The Pathophysiology of Obesity
Introduction
Obesity is a chronic disease manifested as excess adipose tissue. Obesity has multiple etiologies including genetic, environmental, behavioral and defects in neurohormonal signaling.
Energy Balance/Homeostatic Regulation of Food Intake
Energy homeostasis is the steady state balance between energy intake vs energy expenditure, and humans have evolved multiple mechanisms to maintain energy homeostasis. Homeostatic control of food intake involves complex communication between the central nervous system (CNS) (hypothalamus) and the periphery. Intake involves the process of obtaining and digesting nutrients, as well as the regulation of feeding behavior. Energy expenditure involves basal metabolic rate, non-shivering thermogenesis, diet-induced thermogenesis and physical activity. Basal metabolic rate accounts for approximately 60% to 70% of total energy expenditure (TEE) and increases with overall body weight as the demand increases with the increased body mass.
Thermogenesis…
To continue reading
Log in or register to continue reading. It's free!
OR
By signing up to create an account, I accept Healio's Terms of Use and Privacy Policy.
Introduction
Obesity is a chronic disease manifested as excess adipose tissue. Obesity has multiple etiologies including genetic, environmental, behavioral and defects in neurohormonal signaling.
Energy Balance/Homeostatic Regulation of Food Intake
Energy homeostasis is the steady state balance between energy intake vs energy expenditure, and humans have evolved multiple mechanisms to maintain energy homeostasis. Homeostatic control of food intake involves complex communication between the central nervous system (CNS) (hypothalamus) and the periphery. Intake involves the process of obtaining and digesting nutrients, as well as the regulation of feeding behavior. Energy expenditure involves basal metabolic rate, non-shivering thermogenesis, diet-induced thermogenesis and physical activity. Basal metabolic rate accounts for approximately 60% to 70% of total energy expenditure (TEE) and increases with overall body weight as the demand increases with the increased body mass.
Thermogenesis contributes to energy expenditure, including the regulation of brown adipose tissue for heat generation. Further, after eating, the body utilizes energy for digestion and absorption in a process called diet-induced thermogenesis. Lastly, physical activity is responsible for approximately 20% to 30% of total energy expenditure and is one of the most modifiable components of energy expenditure.
In order to maintain balance, a neural regulator (the hypothalamus) senses fuel availability and generates appropriate signals to the neural circuits controlling food intake and energy expenditure, referred to as the homeostatic regulation of adiposity and body weight. Under steady-state conditions, all energy consumed is normally metabolized to maintain basic metabolic rate, thermogenesis and energy expenditure. Excess fuel is stored to be used later and is required for human survival during times of starvation. However, these pathways are now operating under a condition of sustained positive energy balance and the body’s efficient storage of fat can lead to obesity. Ultimately, obesity is a result of a disruption in energy homeostasis.
Hypothalamus as Key Regulator
The hypothalamus is the regulation center of appetite and energy expenditure, integrating both CNS and peripheral signals that subsequently modulate feeding behavior and energy balance. The hypothalamus consists of several interconnecting nuclei, including the arcuate nucleus (ARC), which is considered to be the primary region sensing the peripheral metabolic signals leading to feeding behavior and appetite regulation. Within the ARC, there are two distinct neuronal populations: one which expresses orexigenic peptides including neuropeptide Y (NPY) and agouti-related peptide (AgRP) which functions to reduce energy expenditure and increase appetite (Figure 2-1).
NPY is a 36 amino acid neural transmitter that is widely distributed throughout the CNS with the highest concentration found in the ARC of the hypothalamus. The appetite-stimulating effects of NPY are mediated by several subtypes of NPY receptors on the orexigenic neuron. The production of NPY from the NPY/AgRP neuron is stimulated by the gut “hunger signal” ghrelin and inhibited by leptin, amylin, insulin and serotonin (5-HT).
AgRP is a 132 amino acid peptide signaling molecule co-expressed with NPY in the NPY/AgRP neuron. The production of AgRP from the NPY/AgRP neuron is stimulated by ghrelin and inhibited by leptin, amylin, insulin and 5-HT. AgRP is also highly expressed in the adrenal gland. As an antagonist of α-MSH (melanocyte-stimulating hormone), AgRP competes with α-MSH for binding to the melanocortin receptor 4 (MC4R), leading to lowered satiety and overeating.
The other neuronal population is the anorexigenic peptides, including proopiomelanocortin (POMC) and cocaine- and amphetamine-regulated transcript (CART). POMC is a prohormone synthesized in the POMC/CART neuron in the ARC of the hypothalamus. Activation of POMC neurons leads to release of α-MSH which binds to MC4R, leading to a reduction in appetite and increased energy expenditure. CART is an approximately 50 amino acid long peptide derived in the POMC/CART neuron. Its main function in the hypothalamus is to stimulate anorexigenic neurons to suppress appetite. First discovered as a respondent to cocaine and amphetamine administration, CART is believed to play roles in reward and addiction regulations. Both NPY/AgRP and POMC neurons project from the arcuate nucleus to the hypothalamus (as well as other brain regions), which contains a dense neuronal population that expresses MC4R. Activation of MC4R by α-MSH relays a satiety signal, resulting in a reduction in food intake. This neuronal regulatory system is regulated by modulators such as leptin and insulin (Figure 2-2).
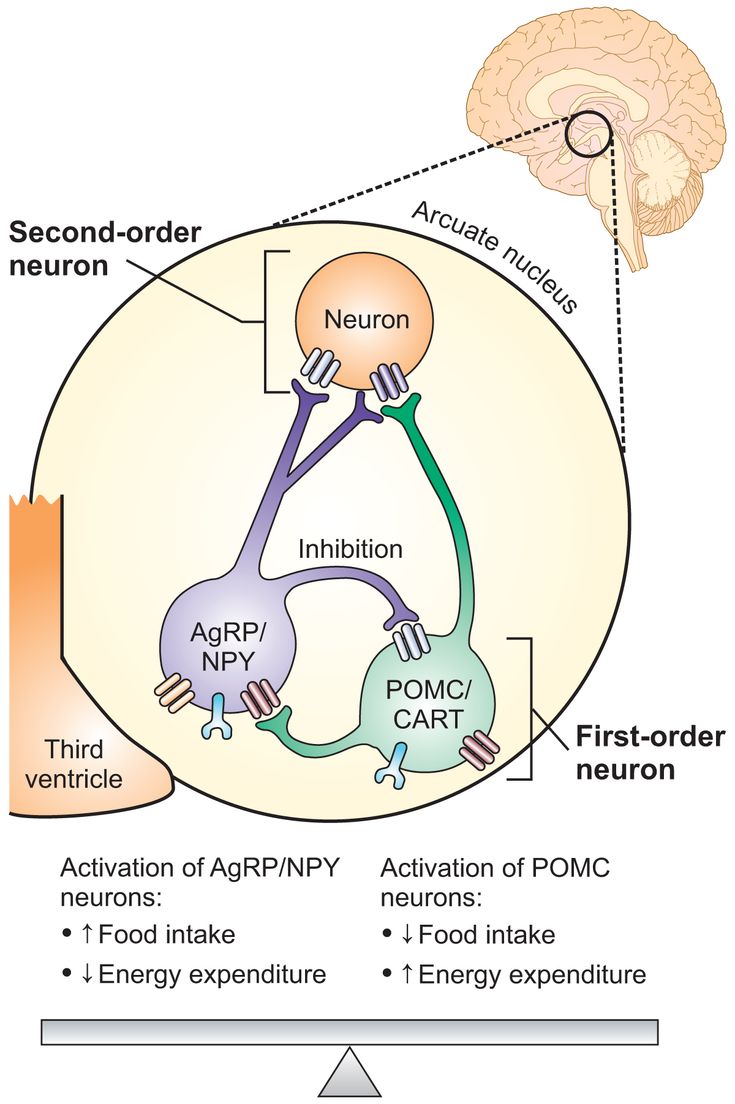
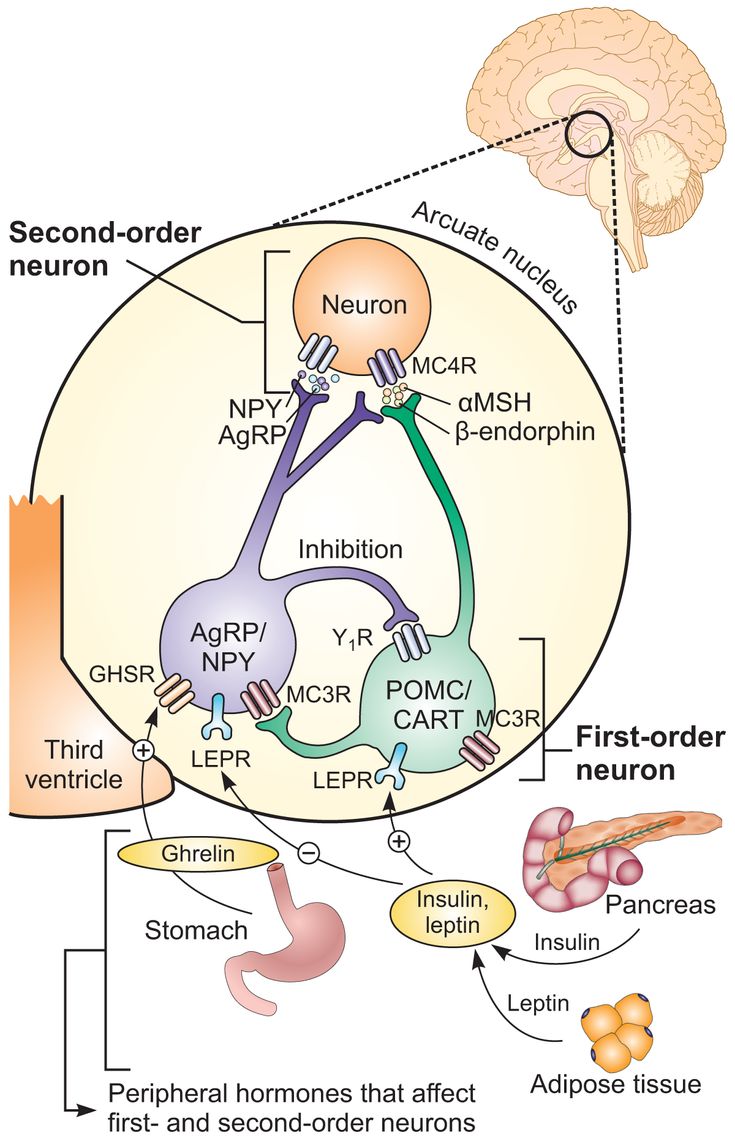
Peripheral Signaling
Peripheral signals send signals to the CNS via three routes:
- Humoral
- Metabolic
- Neural
Humoral factors include hormones secreted by the gastrointestinal (GI) system, adipose tissue and pancreas. These signals include peptides, ghrelin, leptin, insulin, cholecystokinin (CCK) and tumor necrosis factor alpha (TNF-α). Metabolic factors include carbohydrates, lipids ketones and other metabolites. Finally, the autonomic nervous system sends signals from the peripheral organs to the CNS. Subsequently, all of these signals are integrated and regulate both short-term energy intake as well as long-term energy stores to modulate energy intake and energy expenditure. These multiple signaling pathways ensure that food is consumed when needed. However, ongoing access to highly palatable foods may override the inhibitory processes that signal satiety and one may begin to overconsume large amounts of food despite nutrient overload.
Humoral Signaling
Gastrointestinal Signals
The primary role of the GI tract is to digest and absorb nutrients. However, it also plays a role in energy homeostasis via mechanoreceptors and chemosensors which detect the amount and quality of food intake. Gastric distension leads to vagal stimulation due to secretion of serotonin from gastric enterochromaffin cells or from direct stimulation of stretch receptors. The small intestine secretes satiety signals including CCK, peptide YY (PYY), serotonin and glucagon-like peptide-1 (GLP-1) (Figure 2-3).
CCK is released from the small intestine (duodenum and jejunum) in response to fat and protein intake and signals satiety. PYY (and GLP-1 are both released in the small intestine in response to luminal nutrient stimulation). PYY binds to and inactivates NPY/AgRP leading to anorexia. GLP-1 delays gastric emptying leading to improved satiety, reduces hunger and enhances glycemic control.
Ghrelin, secreted from the stomach, exerts an orexigenic effect. Ghrelin levels are elevated during the fasting state and thus is considered the physiologic “hunger” hormone. Ghrelin levels rise before each meal and rapidly fall after eating. Further, diet-induced weight loss in individuals with obesity show increased plasma ghrelin levels, suggesting that ghrelin may represent a compensatory response to altered energy metabolism.
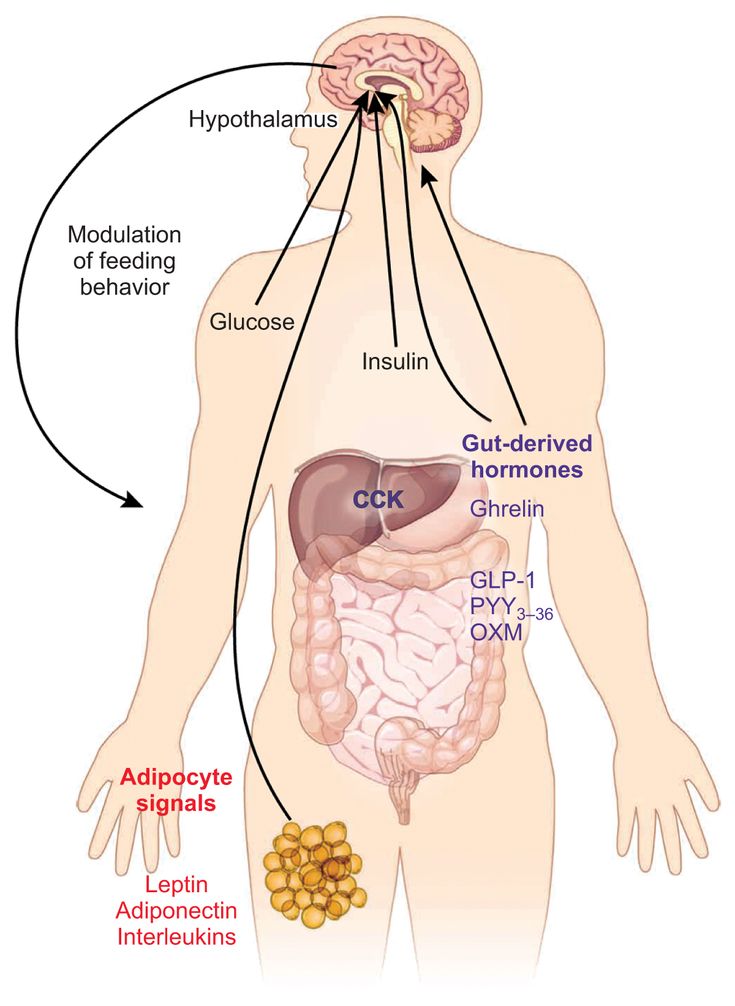
Adipose Signals
Adipose tissue has been recognized as more than just a depot of excess fat. Adipose tissue is recognized as an active organ that secretes a variety of hormones and adipokines, all of which act on a variety of metabolic processes and influence energy homeostasis. Key signaling molecules include leptin, insulin, TNF-α, IL-6 and resistin (Figure 2-3).
Leptin is a hormone discovered in 1994 which is secreted by adipose tissue and in normal individuals, leptin levels correlate with adipose tissue mass. Leptin receptors are highly expressed in the ARC of the hypothalamus. Binding of the leptin receptors induces an increase in anorexigenic POMC/CART signaling and decreased activity of the orexigenic signals NPY/AgRP, resulting in reduced food intake and increased energy expenditure. Studies have shown that leptin acts as a satiety factor that signals the CNS that adipose tissue stores are adequate. The absence of leptin acts as a signal of starvation, thus leptin deficient individuals develop severe obesity and hyperphagia. Leptin has successfully treated hyperphagia in leptin deficient individuals; however, most people with obesity have elevated leptin levels, implying leptin resistance and treatment in these patients has been ineffective.
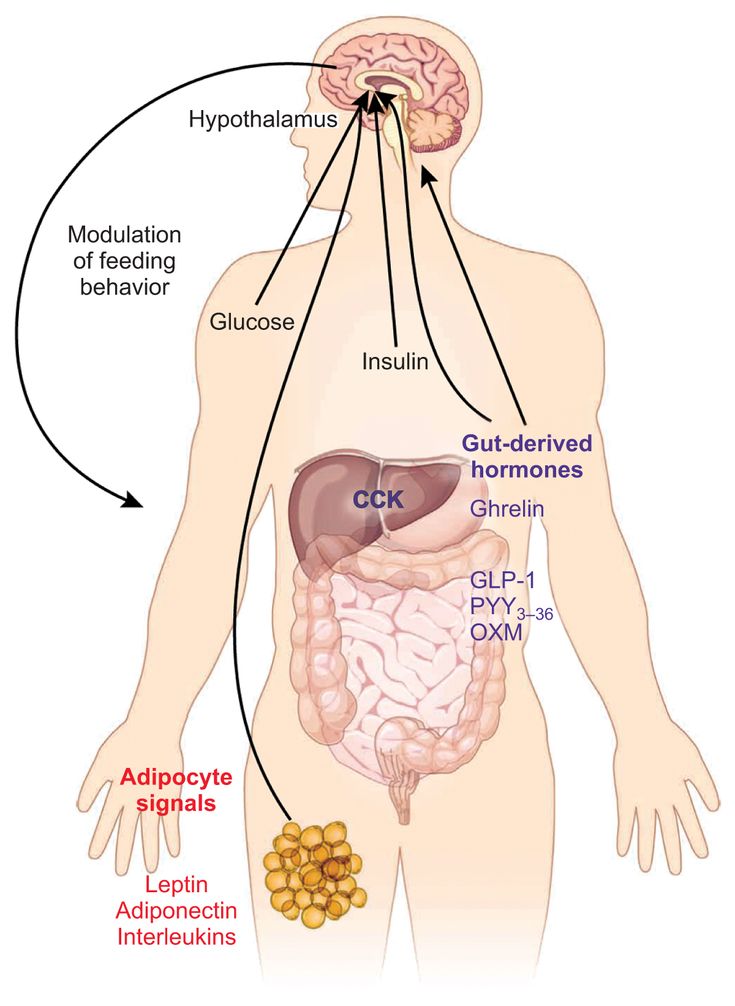
Pancreatic Signals
Insulin is secreted from the pancreatic β cells following a meal and transported to the brain (Figure 2-2). Fasting insulin levels positively correlate with body fat mass and insulin has been considered a surrogate marker for adiposity. Insulin receptors are expressed in the hypothalamic nuclei including the ARC. Insulin, similar to leptin, binds to the ARC neurons and results in POMC activation and NPY/AgRP inhibition, leading to reduced food intake.
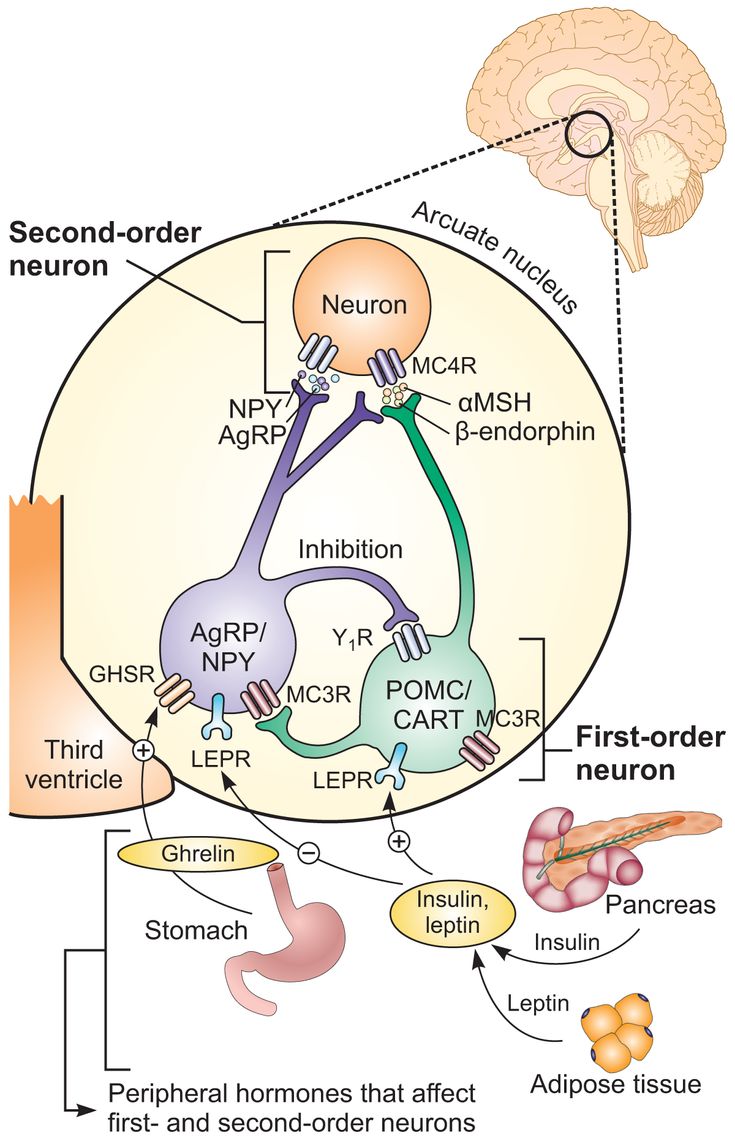
Reward or “Hedonic” Pathway
Certain forms of obesity may be driven by excessive motivational drive for food and mediated by reward “hedonic” circuitry. Certain foods, particularly those containing sugar and fat, are potently rewarding. In animal models, this can trigger addictive-like behaviors; however, the response to food by humans is more complex. In humans, the rewarding property of food is influenced by many other factors including palatability, availability, economics and incentives (“supersizing”) and social routines. During periods of energy abundance, the reward system regulation can override the homeostatic pathway (by increasing the desire to consume foods that are highly palatable), leading to obesity.
Several neurotransmitters have been implicated in the rewarding effect of food; however, dopamine has been the most thoroughly investigated and is best characterized. Upon exposure to a food reward, dopamine neurons fire, leading to an increase in dopamine release in the nucleus accumbens (NAc). Disruption of the dopamine reward pathway has been implicated in the loss of control seen in obesity (Figure 2-4).
In addition, individuals with obesity may respond to food differently than their unaffected counterparts. Data using functional magnetic resonance imaging (MRI) have demonstrated that high glycemic index meals (highly palatable foods) increase activity in the NAc. Furthermore, functional MRI shows significantly greater activation of the NAc in women with obesity compared with women of normal weight. This greater activation was observed in response to pictures of high-calorie (e.g., cheesecake, ribs) vs low-calorie foods (e.g., steamed vegetables, broiled fish). Exaggerated reactivity to food cues, especially those associated with high-calorie foods, may be a factor underlying obesity. This increased motivational potency of foods in individuals with obesity appears to be mediated in part by a hyperactive reward system.
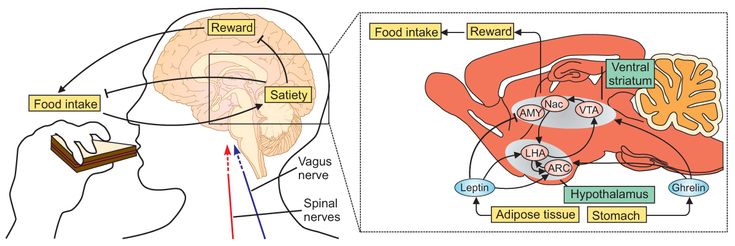
Role of Obesity Pharmacotherapy
The current approved antiobesity pharmacotherapy targets the above pathways in an effort to manage appetite and reduce weight. Phentermine increases dopamine and norepinephrine in the hypothalamus enhancing POMC neuron pathways to increase alpha-MSH, which binds to MC4R to partially suppress appetite. Bupropion SR plus naltrexone SR targets the POMC pathway. Bupropion may enhance POMC-mediated appetite suppression; however, it also activates the β-endorphin/opioid-mediated negative feedback loop which mitigates how much bupropion can activate POMC. Thus naltrexone removes this negative feedback and can potentiate bupropion’s ability to increase POMC firing, leading to stronger appetite suppression (Figure 2-5). Two additional therapeutic agents, liraglutide and semaglutide, are GLP-1 receptor agonists. They affect multiple pathways and organs to reduce appetite, energy intake and hunger and promote satiety (Figure 2-6).
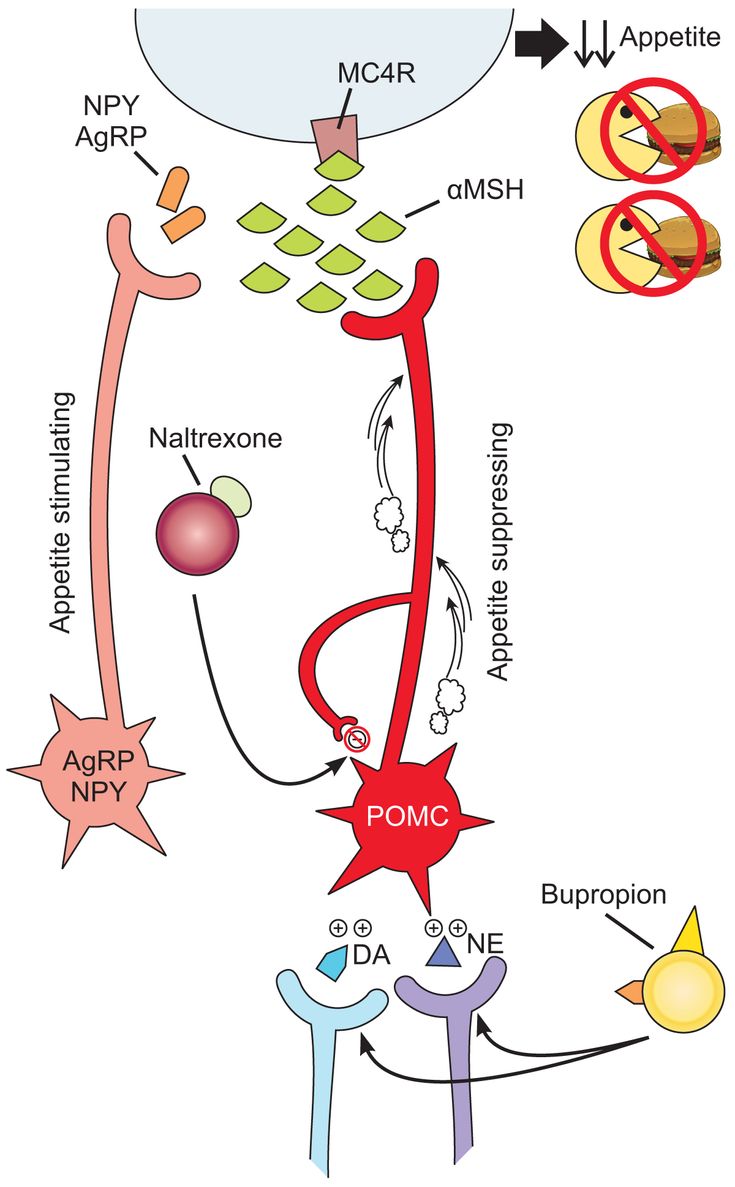
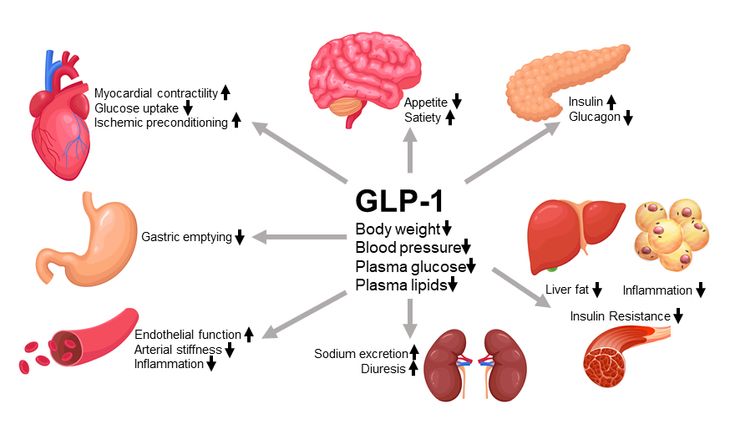
Genetic and Environmental Factors
Genetics
Genetic causes of obesity may derive from monogenic or polygenic hypothalamic defects resulting in impairment in the ability of the hypothalamic circuitry to regulate body weight by controlling energy expenditure, food intake and some peripheral metabolic actions. The latest version of the human obesity gene map reported 11 human genes that cause monogenic obesity and 52 genomic regions harboring a trait loci associated with obesity. Single gene mutations can result in syndromes in which obesity is a symptom; including Leptin Deficiency, Leptin Receptor Deficiency, Prader-Willi Syndrome and Bardet-Biedl syndrome and mutations in the FTO, POMC, and MC4R genes.
Leptin deficiency is a mutation of the LEP gene which encodes for leptin. It is associated with class III, early-onset obesity and was the first monogenic form of obesity discovered. Carriers of leptin gene mutations are able to normalize their body weight after daily subcutaneous leptin administration. Leptin receptor deficiency is a rare autosomal recessive condition that occurs due to mutations in the leptin receptor (LEPR) gene. Along with obesity, leptin receptor deficiency can lead to hypogonadotropic hypogonadism leading to delayed sexual development and infertility. The Prader-Willi syndrome is a neurodegenerative disorder that is caused by genetic abnormalities of the long arm of chromosome 15 (q11-13). Affected infants have poor muscle tone and feed poorly at birth. Later their appetite greatly increases leading to hyperphagia and obesity. They also have behavior problems (irritability, tantrums), delayed development, short stature, and, later, hypogonadotropic hypogonadism. Bardet-Biedl syndrome occurs from mutations to the primary cilium altering cellular signaling and is a disorder characterized by obesity and several other abnormalities, including microorchidism in men, intellectual disability (mental retardation), retinal dystrophy, polydactyly, renal malformations (particularly calyceal abnormalities) and polyuria and polydipsia.
Humans with the two copies of the FTO gene (fat mass and obesity associated gene) have been found on average to weight 3 to 4 kg more and have a 1.67-fold greater risk of obesity compared with those without the risk allele. In addition, human studies have found that both adults and children with at least one FTO risk allele report greater food intake, impaired satiety responsiveness and more frequent eating loss of control. The increased consumed energy was due to an increased preference for energy dense foods, specifically those with a higher fat content. Furthermore, FTO is strongly expressed in the hypothalamus, particularly the arcuate, paraventricular (PVN), dorsomedial and ventromedial nuclei, all key regions crucial to energy intake.
POMC deficiency is characterized by severe, early-onset hyperphagic obesity and congenital adrenal insufficiency. In the first months of life, most children with POMC deficiency experience exponential weight gain, hyperphagia, cholestasis and adrenal insufficiency. Weight gain continues rapidly so that by the end of the first year of life, obesity is severe.
MC4R gene deficiency is the most common form of monogenic obesity with general prevalence of 1-5% in patients with obesity. Pathogenic mutations in MC4R cause low MC4R functionality and impact the leptin-melanocortin signaling pathway. The deficiency is characterized by early-onset obesity, hyperphagia, increased linear growth and, interestingly, a lower tendency for developing hypertension. It is typically inherited in an autosomal (co-)dominant pattern, although penetrance is not always complete. Homozygous MC4R genotypes are much rarer than heterozygous genotypes, but are associated with more severe obesity.
There are several additional genetic and syndromic causes of obesity; however, they are rare and outside of the scope of this handbook.
Epigenetics
Among the different mechanisms that can lead to interindividual differences in obesity, the epigenetic regulation of gene expression has emerged in the past few years as a potentially important contributor. Epigenetics are heritable changes in gene activity which are not caused by changes in the DNA sequence itself. Epigenetic mechanisms are intrinsically malleable and can be influenced by factors including diet, pharmacologic agents and environmental toxins.
As an example, the fetus or neonate is extremely sensitive to perturbation by chemicals with hormone-like activity. Environmental chemicals can disrupt the programming of endocrine signaling pathways that are established during perinatal life and result in adverse consequences into adulthood. These endocrine disruptors include pesticides, bisphenol A, organophosphates, polychlorinated biphenyls, polybrominated biphenyls, phthalates and heavy metals. As an example, in utero or neonatal exposure to Bisphenol A may interact with other factors that influence fetal and postnatal growth in contributing to the obesity epidemic.
Furthermore, studies have examined the intrauterine environment of women with obesity to understand whether it induces developmental adaptations in the developing fetus that then predispose that fetus to obesity. This has been demonstrated in data from women with obesity who underwent bariatric surgery. The children born after maternal weight loss have a lower risk of developing obesity than do their siblings born before maternal weight loss. Such “metabolic imprinting” of body weight regulation could occur via epigenetic mechanisms.
Environmental Factors
Environmental influences, including the physical, social and economic environment, have likely all contributed to the obesity epidemic. The physical environment includes easy access/use of automobiles, as well as exposure to pollutants and “obesogens.” The social environment includes recreational eating (social eating influences meal duration and consumption norms), ongoing advertisements of unhealthy foods and availability of larger portion sizes.
Genetics vs Environment
The contribution of genetics and environment to the etiology of obesity has been evaluated by multiple studies. Twin studies have shown that genetics explain 50% to 90% of the variation in BMI. In a study of same-age, unrelated siblings reared together since infancy, 61% of the variance was genetic, 25% due to the common or shared environment and 14% due to the unique environment. Thus genetics likely accounts for 60% to 70% of BMI, whereas environmental factors may explain the remaining 30% to 40%.
In a given population, a person may be genetically susceptible to obesity but only unless exposed to certain environmental conditions, such as a readily available, highly-caloric, high-fat diet and sedentary lifestyle, would it be expressed. Environmental conditions in developing countries favor the genetically susceptible towards obesity. Evidence of this comes from immigrants who move to the United States who show marked differences in the incidence of obesity compared with their counterparts who remain in their native countries. In addition, studies of the Native American Pima people have shown that those residing in Arizona have highest prevalence of obesity vs those living in a traditional lifestyle in remote area of Mexico—(BMI was 24.9 vs 33.4). The groups differ in diet and energy expenditure based on location in which they live and affluence. Those living in Mexico have a diet with lower animal fat and reduced caloric intake vs those in Arizona have higher fat and more calorically dense food with more complex carbohydrates.
Gut Microbes
The human gut is populated with both symbiotic and commensal microbes and there is increasing evidence that the gut microbiota may play a role in the development of obesity. Studies in mice have shown that obesity can be induced in lean mice via fecal transplants from mice with obesity.
The exact mechanism of how gut microbes influence weight is unknown; however, animal models suggest that obesity is associated with alterations in the composition and functional properties of the gut microbiota. Although the data is conflicting, some data suggest a shift in the abundance of two dominating divisions of the bacteria, Bacteroidetes and Firmicutes. Compared with lean individuals, individuals with obesity have a lower ratio from the phylum Bacteroidetes to that of the phylum Firmicutes. Host bacteria may affect energy balance through several mechanisms, including increased fermentation of undigested polysaccharides and obtaining extra energy from the portion of food, reduced expression of fasting-induced adipocyte factor with inhibitory activity towards lipoprotein lipase and increased release of peptide YY which slows intestinal motility.
The gut microbiome (GM) of patients with obesity also changes in response to medical interventions. Patients with class III obesity who are candidates for bariatric surgery exhibit micronutrient deficiencies and dysbiosis. The two are interdependent as microbes produce micronutrients while micronutrients are required for bacterial survival. Changes in gut microbiota following bariatric surgery have been reported. Multiple factors, including anatomical rearrangement of the gut, weight loss, diet, biliary acids and hormones all contribute to sustained changes of the GM. Although some studies report favorable changes to the microbiota following bariatric surgery, others point to deleterious consequences. Administration of probiotics could be considered following these procedures to restore the GM, but more studies are needed.
Furthermore, the key importance of antibiotic use and dietary nutrient composition are increasingly recognized. The role of the Western diet in promoting an obesogenic gut microbiota has been evaluated and shown that it may increase the abundance of Firmicutes at the expense of Bacteroidetes, inducing enrichment in genes enabling energy harvest from the diet. Further, the changes in the microbial composition were completely reversed after a shift back to the original diet.
Medical Conditions
Medical conditions linked to obesity including Cushing’s syndrome, hypothyroidism, PCOS and growth hormone deficiency. Psychiatric conditions may also play a role including binge-eating and night-eating disorders. Finally, multiple medications may contribute to obesity.
Cushing's Syndrome
Cushing’s syndrome describes the signs and symptoms associated with prolonged exposure to inappropriately high levels of the hormone cortisol. This can be caused by taking glucocorticoid drugs, or diseases that result in excess cortisol, adrenocorticotropic hormone (ACTH), or corticotropin-releasing hormone (CRH) levels. Progressive weight gain is the most common symptom of Cushing’s syndrome. This weight gain usually affects the face, neck, trunk and abdomen more than the limbs, which may be thin. People with Cushing’s syndrome often develop a rounded face and collections of fat on the upper back and at the base of the neck.
Hypothyroidism
The relationship between thyroid dysfunction and obesity is complex and bidirectional. Patients with hypothyroidism often gain weight due to slowing of metabolic activity. The weight gain is usually modest and marked obesity is uncommon. Increasing serum thyroid-stimulating hormone (TSH) concentrations within the normal range have also been associated with a modest increase in body weight in adults but treatment of subclinical hypothyroidism does not appear to be associated with weight loss.
PCOS
PCOS is clinically characterized by oligomenorrhea and hyperandrogenism, as well as the frequent presence of obesity, glucose intolerance and dyslipidemia. At least half of all women with PCOS have obesity; however, the relationship between obesity and PCOS is not causal.
Growth Hormone Deficiency
Growth hormone deficiency is associated with weight gain and alterations in body composition, specifically central adiposity and a reduction in lean body mass.
Binge-Eating Disorder
Binge-eating disorder is a psychiatric illness characterized by uncontrolled episodes of eating that usually occur in the evening. During such binges, a person rapidly consumes an excessive amount of food. Most people who have eating binges try to hide this behavior from others and often feel ashamed about having overweight or depressed about their overeating.
Night-Eating Syndrome
Night-eating syndrome is defined as consumption of at least 25% (and usually more than 50%) of energy between the evening meal and the next morning. It is a well-known pattern of disturbed eating that affects approximately 10% of individuals with obesity.
Sleep
Sleep deprivation has been linked to obesity. Sleep is an important modulator of neuroendocrine function and glucose metabolism. Sleep loss has been shown to result in metabolic and endocrine alterations, including decreased glucose tolerance, decreased insulin sensitivity, increased evening concentrations of cortisol, increased levels of ghrelin, decreased levels of leptin and increased hunger and appetite. Studies have shown a correlation between chronic short sleep (6 hours or less) and elevated BMI and waist circumference. Short sleepers were as much as 1.7 kg/m2 heavier and waist 3.4 cm greater than long sleepers (>10 hours).
Hypothalamic Obesity
Hypothalamic obesity (HO) comprises a series of genetic or acquired pathologic processes damaging the hypothalamic centers of body weight and energy expenditure leading to obesity. Specifically, HO is generally associated with damage to the ventromedial hypothalamus leading to hyperphagia, autonomic dysfunction and decreased energy expenditure. One of the first hypothalamic syndromes described was Babinski-Frohlich syndrome or hypothalamic infantilism obesity whereby a pituitary tumor led to a disorder characterized by headaches, visual changes, obesity and hypogonadism, which is now known to be due to hypopituitarism. It is now understood that structural damage to the hypothalamus can lead to obesity including neoplasms (e.g., craniopharyngiomas), vascular malformations and inflammatory or infiltrative diseases.
Adaptive Responses and Hormonal Changes to Weight Loss
Weight loss itself is difficult for most patients; however, maintaining the weight loss can be even more challenging. There are compensatory changes that occur with weight loss which may promote weight regain due to decreased daily resting energy expenditure (REE) and changes in peripheral signals that affect appetite stimulation and suppression.
Weight loss is associated with a reduction in TEE that is out of proportion to changes in lean body mass, the primary determinant of resting energy expenditure. Liebel and colleagues evaluated 18 subjects with obesity vs 23 subjects who had never had obesity. They were studied at their usual body weight and after losing 10% to 20% of their weight by underfeeding. The 24-hour energy expenditure, resting and nonresting, were evaluated. Results demonstrated that maintenance of a body weight at a level 10% or more below the initial weight was associated with a mean reduction in TEE of 6 ± 3 kcal per kilogram of fat-free mass per day in subjects who never had obesity (P <0.001) and 8 ± 5 kcal per kilogram per day in subjects with obesity (P <0.001). REE and non-REE each decreased 3 to 4 kcal per kilogram of fat-free mass per day in both groups of subjects. The thermic effect of feeding and non-REE increased by approximately 1 to 2 and 8 to 9 kcal per kilogram of fat-free mass per day, respectively, after weight gain. When adjusted for body composition, a 10% decrease in body weight resulted in 15% lower energy expenditure, which the authors note is substantial considering that an intake of 2,500 kcal (an average daily intake) would result in a positive energy balance of 375 kcal. Thus, maintenance of a reduced weight was associated with compensatory changes in energy expenditure which oppose the maintenance of a body weight that is different from the usual weight.
These compensatory changes may account for the challenges in achieving long-term weight loss success. This reduction in TEE appears to persist indefinitely as long as the reduced weight is maintained. The lower TEE is important in that it means that the individual will need to restrict energy intake indefinitely or regain the lost weight.
Furthermore, weight loss is also associated with an increase in the drive to eat and a reduction in satiety. Increased hunger and decreased satiety following weight loss are associated with increases in the 24-hour profile of circulating levels of the orexigenic hormone ghrelin, and reductions in the levels of the anorexigenic hormones PYY, CCK, leptin and insulin. These changes in appetite-related hormones appear to persist for at least 1 year following weight reduction and may remain altered indefinitely in a manner that promotes weight regain.
Summary
Obesity is a disease with a complex etiology. Food intake is regulated by two complementary drives, the homeostatic and hedonic pathways. The homeostatic pathway controls energy balance by increasing the motivation to eat following depletion of energy stores. In contrast, hedonic or reward-based regulation can override the homeostatic pathway during periods of relative energy abundance by increasing the desire to consume foods that are highly palatable, subsequently leading to obesity. In addition, there are multiple factors which may contribute to a person’s specific risk for developing obesity, including genetic and environmental factors. Adaptive metabolic responses drive weight regain and it is important for patients to understand that the difficulties surrounding weight loss and weight maintenance are not simply a matter of will-power.
References
- Apovian CM, Aronne L, Barenbaum SR. Clinical Management of Obesity. 2nd ed. Professional Communications Inc. 2022
- Beccuti G, Pannain S. Sleep and obesity. Curr Opin Clin Nutr Metab Care. 2011;14(4):402-412.
- Ciobârcă D, Cătoi AF, Copăescu C, Miere D, Crișan G. Bariatric surgery in obesity: effects on gut microbiota and micronutrient status. Nutrients. 2020;12(1):235.
- Collet TH, Dubern B, Mokrosinski J, et al. Evaluation of a melanocortin-4 receptor (MC4R) agonist (Setmelanotide) in MC4R deficiency. Mol Metab. 2017;6(10):1321-1329.
- Cummings DE, Weigle DS, Frayo RS, et al. Plasma ghrelin levels after diet-induced weight loss or gastric bypass surgery. N Engl J Med. 2002;346(21):1623-1630.
- Farooqi IS, Matarese G, Lord GM, et al. Beneficial effects of leptin on obesity, T cell hyporesponsiveness, and neuroendocrine/metabolic dysfunction of human congenital leptin deficiency. J Clin Invest. 2002;110(8):1093-1103.
- Ford ES, Li C, Wheaton AG, Chapman DP, Perry GS, Croft JB. Sleep duration and body mass index and waist circumference among US adults. Obesity (Silver Spring). 2014;22(2):598-607.
- Garber AK, Lustig RH. Is fast food addictive? Curr Drug Abuse Rev. 2011;4(3):146-162.
- Iepsen EW, Zhang J, Thomsen HS, et al. Patients with obesity caused by melanocortin-4 receptor mutations can be treated with a glucagon-like peptide-1 receptor agonist. Cell Metab. 2018;28(1):23-32.e3.
- Jirtle RL, Skinner MK. Environmental epigenomics and disease susceptibility. Nat Rev Genet. 2007;8(4):253-262.
- Kral JG, Biron S, Simard S, et al. Large maternal weight loss from obesity surgery prevents transmission of obesity to children who were followed for 2 to 18 years. Pediatrics. 2006;118(6):e1644-e1649.
- Lee EB, Ahima RS. Central regulation of appetite and satiety behavior. In: Preedy VR, Watson RR, Martin CR, eds. Handbook of Behavior, Food and Nutrition. New York, NY: Springer; 2011:1023-1034.
- Leibel RL, Rosenbaum M, Hirsch J. Changes in energy expenditure resulting from altered body weight. N Engl J Med. 1995;332(10):621-628.
- Lenard NR, Berthoud HR. Central and peripheral regulation of food intake and physical activity: pathways and genes. Obesity (Silver Spring). 2008;16(suppl 3):S11-S22.
- Lennerz BS, Alsop DC, Holsen LM, et al. Effects of dietary glycemic index on brain regions related to reward and craving in men. Am J Clin Nutr. 2013;98(3):641-647.
- Ley RE, Backhed F, Turnbaugh P, Lozupone CA, Knight RD, Gordon JI. Obesity alters gut microbial etiology. Proc Natl Acad Sci USA. 2005;102(31):11070-11075.
- Minokoshi Y, Alquier T, Furukawa N, et al. AMP-kinase regulates food intake by responding to hormonal and nutrient signals in the hypothalamus. Nature. 2004;428(6982):569-574.
- Morton GJ, Meek TH, Schwartz MW. Neurobiology of food intake in health and disease. Nat Rev Neurosci. 2014 ;15(6) :367-378.
- Musso, G, Gambino, R, Cassader M. Obesity, diabetes and gut microbiota: the hygiene hypothesis expanded? Diabetes Care. 2010;33(10):2277-2284.
- Rankinen T, Bouchard C. Genetics of food intake and eating behavior phenotypes in humans. Annu Rev Nutr. 2006 ;26 :413-434.
- Ravussin E, Lillioja S, Anderson TE, Christin L, Bogardus C. Determinants of 24-hour energy expenditure in man. Methods and results using a respiratory chamber. J Clin Invest. 1986;78(6):1568-1578.
- Ravussin E, Valencia ME, Esparza J, Bennett PH, Schulz LO. Effects of a traditional lifestyle on obesity in Pima Indians. Diabetes Care. 1994;17(9):1067-1074.
- Reed ML, Merriam GR, Kargi AY. Adult growth hormone deficiency - benefits, side effects, and risks of growth hormone replacement. Front Endocrinol (Lausanne). 2013;4:64.
- Rondinone CM. Adipocyte-derived hormones, cytokines, and mediators. Endocrine. 2006;29(1):81-90.
- Rosenbaum M, Hirsch J, Gallagher DA, Leibel RL. Long-term persistence of adaptive thermogenesis in subjects who have maintained a reduced body weight. Am J Clin Nutr. 2008; 88(4):906-912.
- Sahu A. Leptin signaling in the hypothalamus: emphasis on energy homeostasis and leptin resistance. Front Neuroendocrinol. 2003;24(4):225-253.
- Segal NL, Allison DB. Twins and virtual twins: bases of relative body weight revisited. Int J Obes Relat Metab Disord. 2002;26(4):437-441.
- Stoeckel LE, Weller RE, Cook EW 3rd, Twieg DB, Knowlton RC, Cox JE. Widespread reward-system activation in obese women in response to pictures of high-calorie foods. Neuroimage. 2008;41(2):636-647.
- Stunkard A, Berkowitz R, Wadden T, Tanrikut C, Reiss E, Young L. Binge eating disorder and the night-eating syndrome. Int J Obes Relat Metab Disord. 1996;20(1):1-6.
- Sumithran P, Prendergast LA, Delbridge E, et al. Long-term persistence of hormonal adaptations to weight loss. N Engl J Med. 2011;365(17):1597-1604.
- Sumithran P, Proietto J. The defense of body weight: a physiological basis for weight regain after weight loss. Clin Sci (Lond). 2013;124(4):231-241.
- Tao YX. The melanocortin-4 receptor: physiology, pharmacology, and pathophysiology. Endocr Rev. 2010;31(4):506-43.
- Timpson NJ, Emmett PM, Frayling TM, et al. The fat mass- and obesity-associated locus and dietary intake in children. Am J Clin Nutr. 2008;88(4):971-978.
- Turnbaugh PJ, Ley RE, Mahowald MA, Magrini V, Mardis ER, Gordon JI. An obesity-associated gut microbiome with increased capacity for energy harvest. Nature. 2006; 444(1722):1027-1031.
- Vetter ML, Faulconbridge LF, Webb VL, Wadden TA. Behavioral and pharmacologic therapies for obesity. Nat Rev Endocrinol. 2010;6(10):578-588.
- Volkow ND, Wang GJ, Tomasi D, Baler RD. Obesity and addiction: neurobiological overlaps. Obes Rev. 2013;14(1):2-18.
- Waterland RA, Garza C. Potential mechanisms of metabolic imprinting that lead to chronic disease. Am J Clin Nutr. 1999; 69(2):179-197.
- Yeo GS, Heisler LK. Unraveling the brain regulation of appetite: lessons from genetics. Nat Neurosci. 2012 ;15(10) :1343-1349
- Yu JH, Kim MS. Molecular mechanisms of appetite regulation. Diabetes Metab J. 2012;36(6):391-398.