Pathogenesis and Pathophysiology
Introduction
Understanding the pathogenesis and pathophysiology of a disease enables the development of new treatment strategies, including targeted and personalized therapies, and increases the opportunities for screening and prevention, or, failing that, early detection and diagnosis. This is a continuing challenge in systemic lupus erythematosus (SLE), a chronic autoimmune disorder whose pathophysiology involves a complex network of dysregulated elements of the immune system, including autoantibodies, inflammatory cytokines and complement activation. In many ways, SLE is a prototype autoimmune disease, with involvement of both innate and adaptive system components in an inappropriate response to the body’s own components – primarily nucleic acids – and the activation of the interferon (IFN) system. Despite great advances in recent decades, the pathogenesis and pathophysiology of SLE, above all the etiology and causal hierarchy of immune system dysfunction, remain…
To continue reading
Log in or register to continue reading. It's free!
OR
By signing up to create an account, I accept Healio's Terms of Use and Privacy Policy.
Introduction
Understanding the pathogenesis and pathophysiology of a disease enables the development of new treatment strategies, including targeted and personalized therapies, and increases the opportunities for screening and prevention, or, failing that, early detection and diagnosis. This is a continuing challenge in systemic lupus erythematosus (SLE), a chronic autoimmune disorder whose pathophysiology involves a complex network of dysregulated elements of the immune system, including autoantibodies, inflammatory cytokines and complement activation. In many ways, SLE is a prototype autoimmune disease, with involvement of both innate and adaptive system components in an inappropriate response to the body’s own components – primarily nucleic acids – and the activation of the interferon (IFN) system. Despite great advances in recent decades, the pathogenesis and pathophysiology of SLE, above all the etiology and causal hierarchy of immune system dysfunction, remain poorly understood.
An overview of immune system dysfunction in SLE is shown in Figure 4-1. Immune dysregulation and loss of self-tolerance in SLE arises as a consequence of an interaction between the “exposome” (a sum of environmental influences), the genome (predisposing genetic variants), hormonal factors and stochastic (i.e., randomly determined) factors. Once triggered, the disease follows an unpredictable course, waxing and waning over time, often causing cumulative damages to the tissues and organs in which the aberrant immune complexes accumulate. In this module, we provide an overview of the risk factors for the development of SLE, and discuss the known mechanisms of immune system dysfunction which characterize this disease.
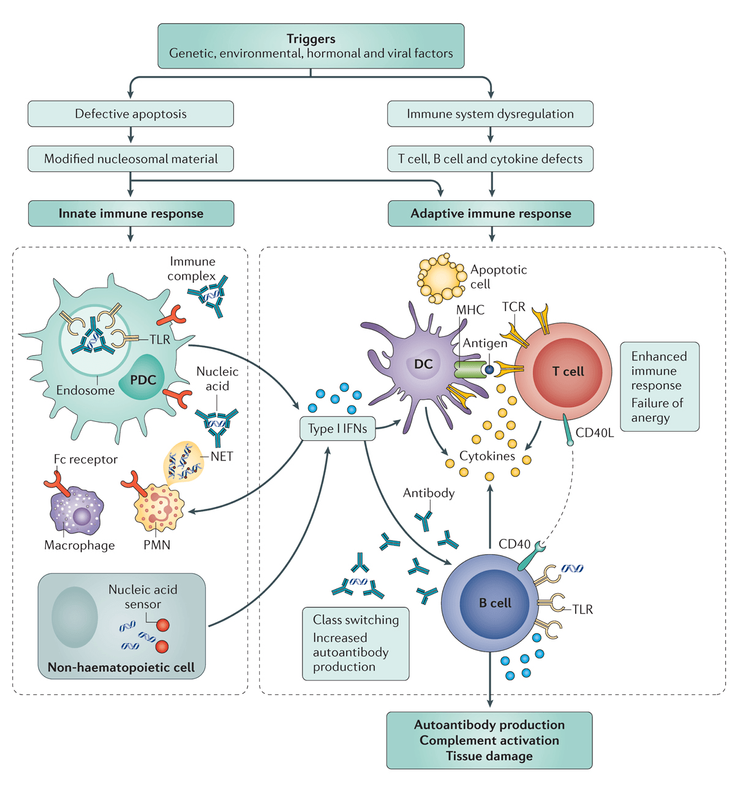
Genetic and Epigenetic Risk Factors
Genetic factors play a crucial role in the pathogenesis of SLE. The disease has high heritability (44%), 20-fold increased risk in siblings of affected individuals and 10-fold higher risk in monozygotic twins compared to dizygotic twins, with a concordance of ~30%.
In the majority of cases, SLE is characterized by polygenic inheritance, with alleles in a large number of loci acting collectively to increase disease susceptibility. More than 700 polymorphisms have been associated with SLE, of which 21 are known to cause amino acid substitutions, nearly 500 are in coding regions but do not affect the protein sequence and more than 200 are in intergenic regions and presumably involved in transcriptional regulation. In some genes (e.g., C4 or TLRs), SLE susceptibility seems to be mediated by copy number variation rather than coding mutations. Genes with variants reliably linked to SLE development are shown in Table 4-1. Most of these encode proteins known to be involved in pathways associated with SLE pathogenesis, including apoptosis, antigen presentation, complement and immune complex clearance, and lymphocyte and phagocyte function; for some, however, the link to immune dysregulation is less clear. The challenge of the coming years will be to molecularly test the candidates identified in genetic association studies, link the individual variants to disease states if possible, and attempt to clinically translate the genetic findings.
Although SLE is typically polygenic, there also exist rare variant which may cause a lupus-like disease which is inherited in a Mendelian fashion. This monogenic lupus is characterized by an early onset, unusually severe manifestations, a strong family history of disease and often, consanguinity. More than 30 genes have been associated with monogenic lupus, including complement components (e.g., C1q, C1r, etc.), genes involved in DNA damage repair and clearance (e.g., DNASE1L3, TREX1) and regulators of IFN genes (TMEM173, IFIH1), among others. The TLR7Y264H gain-of-function mutation, first described in 2022 as a de-novo mutation in a child with severe SLE, was shown to be causative of autoimmunity in mice.
Epigenetics – the study of chromosomal structural changes that alter transcription without affecting the DNA sequence – represents an important mechanism of interaction between genetic susceptibility loci and environmental triggers. Epigenetic alterations that have been reported in SLE include changes in DNA methylation (e.g., hypomethylation in CD4+ T cell and B cells of loci encoding inflammatory cytokines), histone deacetylation and altered micro RNA (miRNA) expression (e.g., lower levels of the miRNA targeting IFNa and IFNb, miR-146a, are correlated with increased SLE disease activity). At present, our understanding of epigenetic mechanisms involved in SLE pathogenesis and pathophysiology remains rudimentary.
Environmental Risk Factors
With the possible exception of monogenic lupus, for SLE to develop, genetic risk factors must interact with environmental triggers, including biologic (infections, microbiome perturbations), physical (UV radiation) and/or chemical (various exogenous toxins and drugs) factors. The exposome modulates the immune response, for example activating Toll-like receptors (TLRs) or modifying self-antigens.
Systemic LE has long been associated with infectious agents, including viruses (most notably the Epstein-Barr virus) and bacteria (most notably Mycoplasma pneumoniae). Chronic viral infections have striking parallels to SLE, with increased type I IFN signaling, pro-inflammatory cytokine (IL-6, IL-10, TNF) signaling, T-cell dysregulation, B-cell overproliferation, autoantibody production and tissue/organ damage. Infection may lead to immune dysregulation through a number of mechanisms, including molecular mimicry between epitopes of infectious and autoantigens, loss of self-tolerance, epitope spreading (development of reactivity to new epitopes distinct from the infectious epitopes), and bystander T-cell activation. Resident microbiota can also contribute to immune dysregulation through mechanisms similar to that of infectious pathogens; this is particularly true for gut microbiota which may cross the gut barrier and move to other sites (mesenteric lymph nodes, spleen, liver) to promote the proliferation of follicular helper T cells and pro-inflammatory T helper 17 (Th17) cells.
The quintessential physical risk factor for SLE is UV light exposure. Although typically thought of as a trigger for the exacerbation of pre-existing disease (above all cutaneous manifestations), UV light is a potential trigger for disease development as well. It causes DNA damage and induces apoptosis in skin cells, leading to an abundance of autoantigens. It may also affect the distribution of intracellular antigens, bringing SSA/Ro, SSB/La and snRNP complexes (common targets of autoantibodies in SLE) closer to the cell surface in apoptotic cells, thus increasing their exposure to immune cells.
Chemical risk factors for SLE include smoking, environmental toxins and certain medications. There is a dose-response association between the number of tobacco cigarettes smoked and the development of SLE. Although the exact explanation for the increased risk is unknown, systemic inflammation, generation of DNA adducts, oxidative stress, and changes in T-cell and B-cell function have been proposed as possible mechanisms. Other exogenous toxins that have been linked to SLE include silica dust, petroleum, organic solvents, pesticides, cosmetics and mineral oils; the list includes compounds with a wide use in the food, cosmetic and pharmaceutical industries, such as pristane. However, no study has established a clear enough link to justify specific recommendations. Finally, more than 100 medications have been associated with drug-induced lupus (see Section 2 for more information on this type of lupus).
Sex and Hormonal Risk Factors
Systemic LE exhibits an extreme sex skew in prevalence, with 9 affected adult women to every affected adult man. The fact that the skew is non-existent (sex ratio 1:1) in children <5 years of age and less pronounced in older prepubescent children (sex ratio ~3:1) argues for a role for sex hormones in the development of SLE. Estrogen is known to modulate lymphocyte function, altering the thresholds for B-cell apoptosis and activation and promoting T-cell activation. Furthermore, estrogen affects the expression of immunomodulatory cytokines, including IL-1 and IL-17. Hormones likely represent only a part of the explanation for the sex skew in SLE, with X-chromosome-linked factors also contributing. For example, persons with Klinefelter syndrome, who are typically male with a 47XXY karyotype, have a 14-fold increased prevalence of SLE compared to 46XY males.
Innate Immune System Dysregulation
Dysregulation of both the adaptive and the innate immune system is the central feature of SLE pathogenesis. Abnormal apoptosis and impaired clearance of apoptotic cells or their debris is one of the major components of immune dysfunction, since it provides abundant autoantigens for the development of an inappropriate response. Nucleic acids (RNA and DNA) and nucleic acid-bound immune complexes can be bound by Toll-like receptors (TLRs), a diverse class of extracellular and intracellular receptors that recognize exogenous and endogenous antigens. Other receptors, including RIG1, MDA5 and cyclic GMP–AMP synthase (cGAS), can also recognize nucleic acids. The binding of nucleic acids to TLRs or other receptors leads to the production of type I IFNs and upregulates the expression of several pro-inflammatory cytokines – a transcriptional program known as the “IFN signature”. The primary cells that drive production of type I IFN are plasmacytoid dendritic cells (pDCs). However, downstream signal amplification involves other components of the innate immune system, including granulocytes (neutrophils, eosinophils and basophils) and neutrophil extracellular traps (NETs; extracellular complexes of DNA and pro-inflammatory proteins produced by neutrophils). These cells and complexes represent an important node in the inflammatory network of SLE because they feed back into IFN production by pDCs, collect self-antigens for presentation to cells of the adaptive immune system and promote damage to the vasculature. Phagocytosis is another aspect of many innate immune system cells that is often impaired in SLE, commonly due to deficiencies in complement-binding receptors (e.g., CR1) or components of the complement pathway itself.
Interferons, Inflammatory Cytokines, and Chemokines
Cytokines are small polypeptide hormones that mediate, modulate and coordinate interactions between cells of both the innate and the adaptive immune system. More than 200 cytokines have been identified to date, and their signaling network is further complicated by receptor sharing, pleiotropy, redundancy and inter-cytokine synergism or antagonism. As we have seen, type I IFN signaling is upregulated in SLE, with as many as 87% or more of patients exhibiting an “IFN signature” of transcripts associated with immunomodulation. This is driven primarily by pDCs, although other immune cells may also contribute. B cells activated by the action of auto-antigen presenting follicular DCs start producing pro-inflammatory cytokines, including the interleukins (ILs) IL-6, IL-8, IL-1β, IL-10, IL-12 and tumor necrosis factor α (TNF-α). Interleukin-17, produced by Th17 lymphocytes, is another important pro-inflammatory cytokine often elevated in SLE, as are its regulator, IL-23 and the regulator of IL-23, IL-21. By contrast, IL-2 is typically low in patients with SLE; among its many functions in T-cell development, IL-2 promotes the activity of T-regulatory (Treg) cells, which antagonize IL-17 signaling.
T-cell Dysregulation
T cells are a significant component in the network of SLE pathogenesis and pathophysiology. In addition to producing pro-inflammatory cytokines, CD4+ T cells, important mediators of B cell differentiation and activation, show increased activity (and higher expression of T-B cells interaction protein CD40L) in patients with SLE; as noted in the following section, B cells are not passive recipients of activating signals from T cells, but can conversely activate T cells. Although the etiology of altered T cell signaling in SLE is unknown, several abnormalities have been reported, including changes in Fc receptor signaling components (substitution of TCRζ with TCRγ), mitochondrial hyperpolarization, increased production of reactive oxygen species, ATP depletion, aggregation of lipid rafts containing the TCR/CD3 complex and other signaling molecules, and increased intracellular calcium signaling. In addition to molecular changes, T cell population changes represent another pathologic change. The follicular Th cells which promote B cell differentiation and activation increase in number, as do, especially in severe cases, memory CD8+ T cells, whose expansion is associated with a poor prognosis. Proinflammatory cytokine-producing Th17 cells are increased in number, while the immunosuppressive Treg cells are decreased.
B-cell Dysregulation
Because they are responsible for the production of autoantibodies, the hallmark feature of SLE, B cells can be thought of as central to the SLE disease process. As described above, B cells also produce a variety of pro-inflammatory cytokines and also participate in antigen presentation to T cells. B cell differentiation and proliferation is augmented by increased numbers of Th cells, cytokines that promote these processes, including B-cell activating factor (BAFF), and cell-autonomous processes like a lower activation threshold. Pathologic activation is also sustained by the co-engagement of B cell receptors (BCRs) and TLRs, which promotes antibody class switching and feeds back into IFN signaling, promoting IFN production by pDCs.
Autoantibodies
Anti-nuclear autoantibodies (ANAs) are typically considered the quintessential element of SLE pathophysiology – to the extent that the presence of ANAs is considered an essential “entry criterion” for the classification of SLE in the 2019 EULAR/ACR criteria; see Testing for SLE-associated antibodies for a brief overview of testing for SLE-associated antibodies.
Testing for SLE-associated antibodies
Detection of SLE-associated antibodies, primarily ANAs and certain ANA sub-serologies like anti-dsDNA autoantibodies and anti-ENA autoantibodies (see Terminology of SLE-associated autoantibodies for a discussion on SLE-associated antibody nomenclature), is central to the evaluation of patients with suspected or established SLE.
Broadly, two types of autoantibody testing methodologies are in common use: indirect immunofluorescence (IIF) and solid-phase assays.
Indirect immunofluorescence has been in use for 60 years and is still considered the “gold standard” for ANA detection. The IIF procedure is illustrated in the figure below. As shown in Figure 4-3A, serum dilutions from a patient are pipetted on a slide containing tissue culture cells (originally rodent cells, but now typically the HEp-2 cell line). After a wash to remove unbound antibodies, the slide is incubated with secondary (ie, anti-IgG) antibodies conjugated to a fluorescent molecule which can, after a second wash to remove excess secondary antibodies, be visualized or imaged for analysis. The fluorescent signal observed in the cells can adopt several patterns characteristic of specific ANAs, as shown in Figure 4-3B. For example, anti-dsDNA and anti-histone autoantibodies have a homogenous pattern, while anti-Sm, anti-Ro/SSA, anti-La/SSB and other autoantibodies not directed against chromatin (anti-ENA autoantibodies) show a speckled pattern. Samples are generally considered positive when fluorescence is visible in a 1:80 dilution titer, as in the 2019 EULAR/ACR classification criteria. Note that, depending on the specific mixture of ANAs and other autoantibodies in the sample, multiple overlapping patterns may be generated.
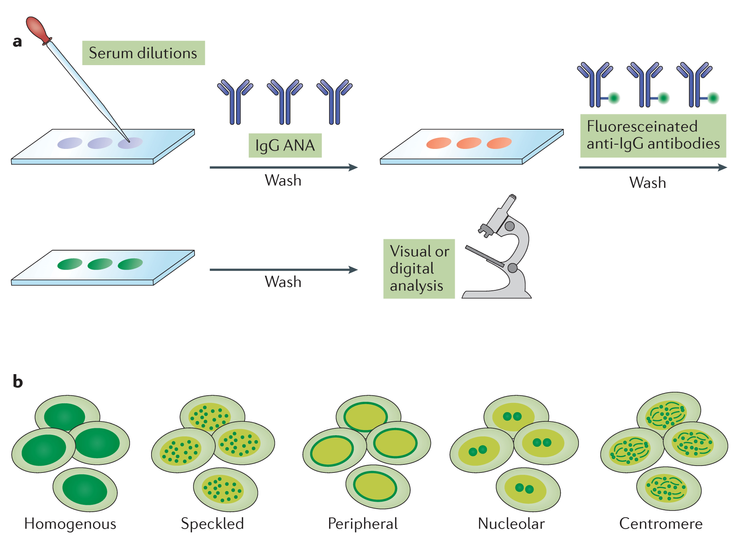
Solid-phase assays dispense with the need for cell culture by using solid surfaces coated with one or more specific autoantigens (e.g., dsDNA or Smith protein). The surfaces are then exposed to patient samples allowing any autoantibodies present in the serum to bind the immobilized autoantigens, following which the surface is washed and incubated with secondary antibodies conjugated to a detection enzyme. Provided with a substrate or oxidizing agent, these enzymes produce a measurable signal – either colorimetric (enzyme-linked immunosorbent assay, or ELISA), fluorescent (fluorometric enzyme-linked immunoassay, or FEIA), or chemiluminescent (chemiluminescence immunoassay, or CIA), from which the concentration of autoantibodies can be calculated.
It is important to note that testing for SLE-associated antibodies is hierarchical, i.e., typically begins by IIF testing for ANAs in general, followed by more specific tests. For example, the 2019 EULAR/ACR classification guidelines require a positive ANA test as an essential entry criterion but have anti-dsDNA and anti-Sm (both highly SLE-specific autoantibodies) seropositivity as additional criteria. A positive sub-serology (e.g., anti-dsDNA) in the absence of ANA positivity from the same laboratory represents cause for doubting the accuracy of the assays used. Finally, it is crucial to remember that ANA positivity alone is not enough to classify a patient as having SLE, as many ANA subtypes (eg, anti-Ro/SSA autoantibodies) are not specific to SLE.
Of the thousands of nuclear proteins, only about a hundred or so are known to be ANA targets – predominantly those that form complexes with nucleic acids, including both DNA and RNA. The skew towards proteins associated with nucleic acids is thought to be a consequence of the immunogenic interaction between DNA/RNA and TLRs in the innate immune system, in a way restricting the pool of potential autoantigens. The major ANAs associated with SLE and their targets are shown in Table 4-2. Anti-dsDNA and anti-Sm antibodies are the most characteristic in SLE. In addition to ANAs proper (see Terminology of SLE-associated autoantibodies), SLE-associated antibodies include those directed against other antigens, including β2 glycoprotein 1 in association with phospholipids (more common in anti-phospholipid syndrome but often present in SLE) and other cell-membrane proteins, and components of the complement system such as C1q.
Terminology of SLE-associated autoantibodies
The first SLE-associated autoantibodies were discovered in the 1950s and were shown to target dsDNA and histones – macromolecular components of the nucleus. Therefore, the term “anti-nuclear autoantibody”, or ANA, was coined in 1959 to describe them. Since then, antibodies to many other nuclear and even non-nuclear (cytoplasmic or cell membrane-bound) antigens have been discovered in patients with SLE, raising the question of whether the label “ANA” has become anachronistic and misleading – at least when understood as a synonym of SLE-associated autoantibody. Several alternatives have been proposed – including “anticellular antibodies” and “antibodies to intracellular antigens” – but none have thus far found widespread acceptance. A new nomenclature should also precisely define which antibodies are included in (each) new term. In the current usage, “ANA” is not an unambiguous and precisely defined term.
Autoantibodies that are always considered ANAs are chromatin-reacting antibodies, including anti-histone and anti-dsDNA antibodies. However, it is important to note that anti-dsDNA antibody titers are often specifically measured in addition to general ANA titers because of their high specificity for SLE. Another sub-class of ANAs are antibodies against extractable nuclear antigens (ENAs). As the name suggests, ENAs are nuclear proteins that can be isolated by salt extraction. They are not chromatin-associated, and include the Smith protein (Sm), ribonucleoproteins (RNPs), and Robert antigen/Sjögren’s A (Ro/SSA) and Lane antigen/Sjögren’s B (La/SSB). Of the autoantibodies directed against ENA, anti-Sm antibodies are the most specific for SLE; as the name implies, anti-Ro/SSA and anti-La/SSB antibodies are more often associated with Sjögren’s syndrome.
Autoantibodies directed against non-nuclear antigens are also frequently found in patients with SLE. These antibodies include anti-C1q, anti-lipoprotein and anti-endothelial autoantibodies, all of which are not considered ANAs. However, it is important to remember that at least some non-nuclear antigen-directed autoantibodies are often considered ANAs – a major example is anti-Ribosomal P autoantibodies, whose antigen is a cytoplasmic (ribosome-associated) protein.
Lupus-associated autoantibodies are known to undergo class-switching from predominantly IgM early in the disease course to IgG or IgA later, which is thought to increase their ability to access non-vascular tissues. This process is promoted by Th cells and various signaling molecules, including TLR ligands, IL-21 and BAFF. Anti-dsDNA antibodies are thought to be produced by circulating plasmablasts, while more protected long-lived plasma cells are thought to be the source of anti-B cell therapy-resistant anti-Sm and anti-Ro antibodies.
Immune Complex Deposition and Tissue/Organ Damage
Autoantibodies complex with their antigen targets to produce immune complexes (ICs), whose deposition in tissues and organs including the skin, kidney and others, is responsible for tissue/organ injury. Not every ANA is pathogenic; ANAs that have been confirmed to cause tissue damage include anti-dsDNA ANAs (associated with lupus nephritis [LN]), antichromatin and antichromatin ANAs (also associated with LN), anti-Ro and anti-La ANAs (associated with cardiac damage in neonatal lupus), anti-N-methyl-D-aspartate receptor antibodies (associated with cognitive changes, memory deficit and neurotoxicity in mouse models), and anti-phospholipid/anti-β2 glycoprotein 1 antibodies (associated with thrombosis). The local apoptosis which results from IC deposition then promotes a positive feedback loop as apoptotic bodies release more autoantigens, promoting further inflammation and IC formation, recruiting lymphocytes and innate immune system cells, all of which results in more tissue damage. Deficient IC clearance plays an important role in this pathway, allowing for pathogenic levels to accumulate in the target tissues. Complement overactivation – by both the classical and the alternative pathway – also feeds into tissue damage by promoting inflammation. Immune complexes and accompanying cytokines can also induce non-apoptotic changes in local cells, including oxidative damage, release of matrix metalloproteases and tissue fibrosis.
A Model of SLE Pathogenesis
The previous sections have offered a brief survey of the various immune defects associated with SLE and how these contribute to the pathology of SLE. While we have a general understanding about which genetic and environmental factors influence the etiology of SLE, many details are still unclear. Above all, a clear chronology of events and their causative hierarchy, ie, which immune system abnormalities are the primary disease drivers and which are secondary or contingent, still eludes us. Our current understanding does allow us to construct a high-level model of the pathogenetic landscape in SLE, as shown in Figure 4-2. Genetic, environmental and hormonal influences impair the clearance of apoptotic cell bodies and complement complexes and drive cells of the innate and adaptive immune system to alter their behavior and promote the inappropriate activation of one another, upregulate the expression of pro-inflammatory cytokines and lose self-antigen tolerance. B cells produce ANAs which, alone or as part of ICs, enter tissues and organs and both cause direct damage and continue the vicious cycle of inflammation and injury, modulated by the tissue/organ microenvironment.
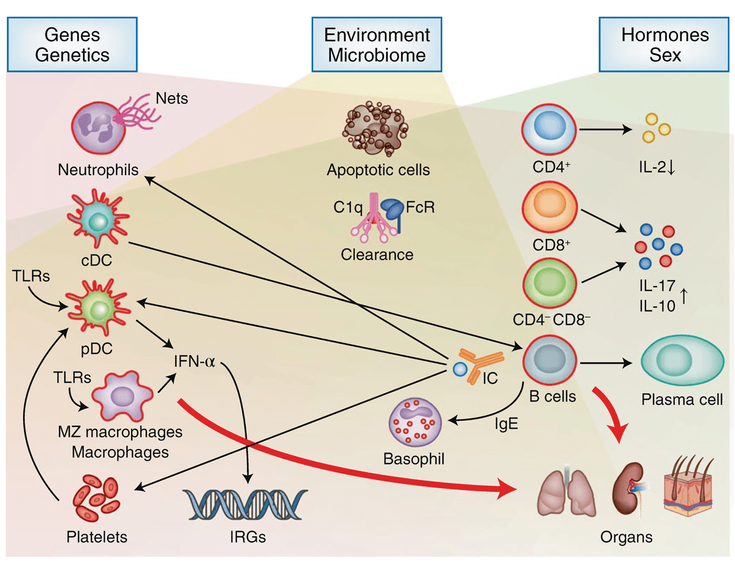
References
- Ameer MA, Chaudhry H, Mushtaq J, et al. An overview of systemic lupus erythematosus (SLE) pathogenesis, classification, and management. Cureus. 2022;14(10):e30330.
- Aringer M, Costenbader K, Daikh D, et al. 2019 European League Against Rheumatism/American College of Rheumatology classification criteria for systemic lupus erythematosus. Ann Rheum Dis. 2019;78(9):1151-1159.
- Arnaud L, Mertz P, Gavand PE, et al. Drug-induced systemic lupus: revisiting the ever-changing spectrum of the disease using the WHO pharmacovigilance database. Ann Rheum Dis. 2019;78(4):504-508.
- Bossuyt X, De Langhe E, Borghi MO, Meroni PL. Understanding and interpreting antinuclear antibody tests in systemic rheumatic diseases. Nat Rev Rheumatol. 2020;16(12):715-726.
- Brown GJ, Canete PF, Wang H, et al. TLR7 gain-of-function genetic variation causes human lupus. Nature. 2022;605:349-356.
- Caielli S, Wan Z, Pascual V. Systemic lupus erythematosus pathogenesis: interferon and beyond. Annu Rev Immunol. 2023;41:533-560.
- Carnero-Montoro E, Barturen G, Alarcón-Riquelme ME. Epigenetics in systemic lupus erythematosus and the integration of molecular pathways. In: Lahita RG. Systemic Lupus Erythematosus. Sixth ed. Amsterdam: Academic Press; 2021:35-61.
- de Leeuw K, Kallenberg CGM. Antibodies Against C1q. In: Wallace DJ, Hahn BH. Dubois' Lupus Erythematosus and Related Syndromes. Ninth ed. Elsevier; 2019:372-374.
- Isenberg D, Giles I, Hansen JE, Rahman A. Antinuclear Antibodies, Antibodies to DNA, Histones, and Nucleosomes. In: Wallace DJ, Hahn BH. Dubois' Lupus Erythematosus and Related Syndromes. Ninth ed. Elsevier; 2019:355-365.
- Katsuyama T, Moulton, VR. Hormones. In: Tsokos GC, ed. Systemic Lupus Erythematosus: Basic Applied and Clinical Aspects. Second ed. London: Academic Press an imprint of Elsevier; 2020:105-112.
- Kaul A, Gordon C, Crow MK, et al. Systemic lupus erythematosus. Nat Rev Dis Primers. 2016;2:16039.
- Kompouras F, Bucala, R. Interferons and cytokines in SLE pathogenesis and therapy. In: Lahita RG. Systemic Lupus Erythematosus. Sixth ed. Amsterdam: Academic Press; 2021:169-180.
- La Cava A. Overview of the pathogenesis of systemic lupus erythematosus. In: Tsokos GC, ed. Systemic Lupus Erythematosus: Basic Applied and Clinical Aspects. Second ed. London: Academic Press an imprint of Elsevier; 2020:69-75.
- Lessard CJ. Lupus susceptibility genes. In: Lahita RG. Systemic Lupus Erythematosus. Sixth ed. Amsterdam: Academic Press; 2021:25-33.
- Lo MS. Monogenic lupus. In: Tsokos GC, ed. Systemic Lupus Erythematosus: Basic Applied and Clinical Aspects. Second ed. London: Academic Press an imprint of Elsevier; 2020:97-104.
- Markewitz RDH. Terminology and definition of ‘antinuclear antibodies’: history and current debate. Ann Rheum Dis. 2022;81:1-2.
- Moulton VR, Suarez-Fueyo A, Meidan E, Li H, Mizui M, Tsokos GC. Pathogenesis of human systemic lupus erythematosus: a cellular perspective. Trends Mol Med. 2017;23(7):615-635.
- Pisetsky DS. Antinuclear antibody testing - misunderstood or misbegotten?. Nat Rev Rheumatol. 2017;13(8):495-502.
- Rahman A. Antilipoprotein and Antiendothelial Cell Antibodies. In: Wallace DJ, Hahn BH. Dubois' Lupus Erythematosus and Related Syndromes. Ninth ed. Elsevier; 2019:375-376.
- Reeves WH, Han S, Zhuang H. Autoantigens, antigenic mimicry, and immune complexes. In: Lahita RG. Systemic Lupus Erythematosus. Sixth ed. Amsterdam: Academic Press; 2021:65-70.
- Riemekasten G, Humrich JY, Hiepe F. Antibodies Against ENA (Sm, RNP, SSA, SSB). In: Wallace DJ, Hahn BH. Dubois' Lupus Erythematosus and Related Syndromes. Ninth ed. Elsevier; 2019:366-371.
- Tsokos GC. Autoimmunity and organ damage in systemic lupus erythematosus. Nat Immunol. 2020;21(6):605-614.
- Yahia SH, Agmon-Levin N. System lupus erythematosus and the environment. In: Tsokos GC, ed. Systemic Lupus Erythematosus: Basic Applied and Clinical Aspects. Second ed. London: Academic Press an imprint of Elsevier; 2020:77-84.