Next-generation sequencing emerges as innovative tool to detect orthopedic infections
Click Here to Manage Email Alerts
Key takeaways:
- Next-generation sequencing continues to outperform culture in diagnosing infections.
- The realm of genomics is ushering in a new era of infection diagnosis.
Next-generation sequencing has emerged as a revolutionary tool with extensive applications in medicine ranging from cancer screening to diagnosing infections across specialties, including orthopedic surgery.
This Infection Watch article delves into the evolution of DNA sequencing, its transition to infection diagnostics and its specific relevance to periprosthetic joint infections.
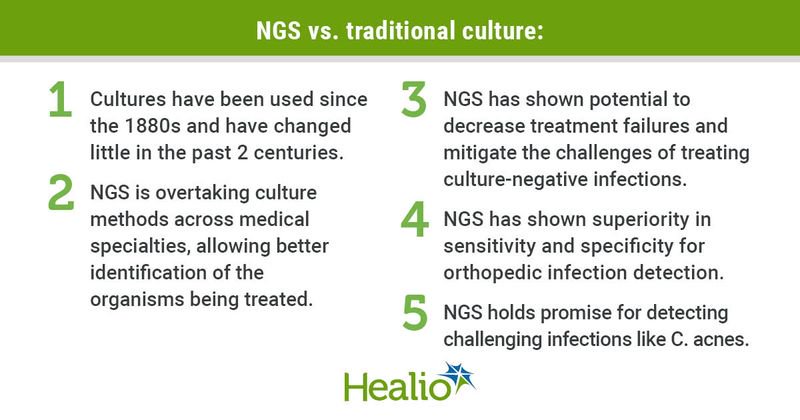
Historical evolution of DNA sequencing
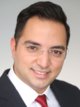
DNA sequencing gained prominence in the 1980s through the Human Genome Project, a monumental endeavor to map every human gene. However, the infectious disease aspect of sequencing began with the parallel sequencing of Haemophilus influenza in 1995. Advancements like automated sequencing and next-generation sequencing (NGS) brought genome sequencing closer to practicality. NGS, also known as massively parallel sequencing, became commercially available in 2005, offering remarkable sensitivity, specificity and affordability.
Challenges with culture-based detection
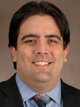
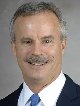
Traditional culture methods have long been the cornerstone of infection detection, but the limitations of these methods, particularly in orthopedic settings, led to the exploration of alternative techniques. Cultures often yield low results, necessitating multiple samples to compensate for this drawback and longer incubation times to grow certain pathogens. Additionally, culture results are influenced by factors such as technique, operator skill and time, posing challenges when dealing with slow-growing organisms like Cutibacterium acnes and Mycobacterium. Variability among labs also raises concerns, as the same samples sent to different facilities can yield disparate outcomes.
Next-generation sequencing
Microbial NGS detects the organisms that may cause infection by quantifying short DNA fragments, which can be thought of as barcodes. These barcode DNA pieces, when sequenced, can be matched against a reference database to learn which bacterium or fungi is a best match. Within a single sample, this process of sequencing a barcode and identifying a microbe can happen thousands of times to quickly quantify the organisms present within a clinical specimen.
From this point, you now have a detailed picture of the identities and proportions of microbes present within a sample and can reference preexisting knowledge of microbe sensitivity to gauge whether adjusting antimicrobial treatment is warranted. Even better, these tests can be paired with quantitative PCR screens to learn about antibiotic resistance-associated genes that may also be found in the sample. Generally, recently published research tends to indicate that treatment fails frequently because of microbes that could have been detected by molecular methods and that patients treated consistent with the microbes detected by NGS have fewer complications caused by infection.
Essential steps
Prior to sequencing, DNA or cDNA (complementary DNA synthesized from RNA) must undergo several essential steps, including fragmentation, end-repair and conversion into sequencing libraries. More simply, sequencing libraries refer to collections of DNA fragments that incorporate adapter sequences tailored for a particular sequencing platform, along with indexing barcodes for individual sample identification. The primary methods for library preparation encompass ligation-based library preparation, tagmentation-based library preparation and amplicon library preparation.
The choice of a specific protocol hinges on the selected sequencing platform and the subsequent analysis requirements. In essence, the fundamental stages of library preparation comprise fragmenting and repairing ends, introducing adapters and optionally performing PCR amplification.
The library is loaded onto a sequencing matrix specific to the chosen sequencer. Various sequencers employ distinct sequencing matrices; for instance, the Illumina NGS sequencer (Novogene) employs flow cells, while the Ion Torrent NGS sequencer (Thermo Fisher Scientific Inc.) utilizes sequencing chips. Despite these variations, the ultimate objective remains consistent: to enable the simultaneous, high-throughput sequencing of targeted regions or all the DNA in the sample.
The sequence data generated are then analyzed bioinformatically. Bioinformatic analysis constitutes a multi-step process encompassing base calling, read alignment and sequence identification. Within this process, the sequenced data are compared against a reference sequence database to identify the organisms from which the DNA originated. All information from each sequenced segment is integrated to produce comprehensive sequencing results spanning the entire length of the targeted DNA or the genomes. These final sequencing results are then provided to the user for interpretation.
NGS vs. traditional culture
Cultures have been used since the 1880s and have changed little in the past 2 centuries. NGS is overtaking culture methods across medical specialties, including orthopedic surgery, to allow for better identification of the organisms being treated.
NGS has shown potential to decrease treatment failures and mitigate the challenges of treating culture-negative infections because of the superiority of NGS in sensitivity and specificity for orthopedic infection detection. A prospective study revealed the ability of NGS to detect signals in culture-negative PJIs, showcasing its potential to identify infections that evade traditional methods. The prowess of NGS is not solely tied to tissue samples; synovial fluid samples from hips and knees yielded infection signals even in culture-negative cases.
This technique also holds promise for detecting challenging infections like C. acnes in shoulder PJIs, known for being difficult to identify without a high index of suspicion.
Polymicrobial infections are a particular challenge in shoulder PJIs. Namadari and colleagues demonstrated that most cultures in their cohort yielded monomicrobial results, whereas NGS could detect polymicrobial infections with a defined load of each organism. With joint aspirations, there often is not enough fluid to be sent for synovial fluid analysis, biomarkers and culture, making culture difficult. Another study found that, even with a low volume of synovial fluid (1 mL), NGS performed with higher sensitivity and specificity compared with synovial culture.
Overcoming challenges, costs
Cost is an important consideration when using diagnostic tools. The cost of a single culture might be lower, nonetheless, multiple samples must be sent to increase the yield. Furthermore, these samples would need to be separately sub-cultured for aerobes, anaerobes, fungi and Mycobacteria.
A cost analysis by Torchia and colleagues found that culture was not cost-effective compared with NGS, with an incremental cost-effectiveness ratio of $422,784 per quality-adjusted life-year. One-way sensitivity analyses found NGS to be the cost-effective choice above a pretest probability of 45.5% for PJI.
Timing is the other important factor for diagnosing PJI. When diagnosed acutely within 3 or 4 weeks, patients can undergo debridement, antibiotics and implant retention (DAIR), rather than a two-stage exchange that could lead to significant morbidity or mortality. Timely diagnosis of PJI can potentially reduce these consequences. While culture often takes 14 to 21 days for slow-growing organisms such as C. acnes, NGS can provide the results within 1 to 3 days. This could improve outcomes and decrease the treatment episode time by at least the difference in the timing to a good diagnosis.
A failure in detecting the infecting organisms can engender a cascade of intricate challenges, leading to an elevated failure rate and a substantial escalation in the economic burden of treatment. Given the intricate nature of these infections, imprecise microbial identification may result in misguided therapeutic interventions, such as empiric administration of broad-spectrum antibiotics, thereby predisposing patients to the emergence of antimicrobial resistance and exacerbation of the infection. Furthermore, delayed, or inaccurate pathogen identification can protract patients' clinical course, amplifying the risk of implant failure and necessitating revision arthroplasty, an intricate procedure associated with heightened morbidity and health care costs.
In essence, the pursuit of meticulous and timely detection of the responsible microorganisms in PJIs stands as a cardinal clinical imperative, serving both as a linchpin for optimized patient care and as a strategic mechanism to curtail the economic repercussions entailed by these intricately challenging cases.
Conclusion
The accessibility and affordability of molecular technologies have propelled clinicians into a transformative phase in infection diagnostics. The superiority of NGS in accuracy, time efficiency and cost-effectiveness positions it as a promising alternative to traditional culture methods. As NGS continues to outperform culture in diagnosing infections, its integration into medical practice is likely to expand, potentially establishing it as the standard of care.
The realm of genomics is ushering in a new era of infection diagnosis, empowering medical professionals with tools that are sensitive, specific and rapid, leading to improved patient outcomes and more efficient treatment strategies.
References:
Fang X, et al. Int J Infect Dis. 2020;doi:10.1016/j.ijid.2020.07.039.
Fink B, et al. Biomed Res Int. 2017;doi:10.1155/2017/4582756.
Fleischmann RD, et al. Science. 1995;doi:10.1126/science.7542800.
Heather JM, et al. Genomics. 2016;doi:10.1016/j.ygeno.2015.11.003.
Hsu JE, et al. J Bone Joint Surg Am. 2016;doi:10.2106/JBJS.15.00568.
Kheir MM, et al. J Arthroplasty. 2018;doi:10.1016/j.arth.2018.06.018.
Miller JM, et al. Clin Infect Dis. 2018;doi:10.1093/cid/ciy381.
Nagendra S, et al. J Clin Microbiol. 2001;doi:10.1128/JCM.39.6.2344-2347.2001.
Namdari S, et al. J Shoulder Elbow Surg. 2019;doi:10.1016/j.jse.2018.08.048.
Shahi A, et al. J Arthroplasty. 2017;doi:10.1016/j.arth.2016.09.027.
Tarabichi M, et al. Bone Joint J. 2018;doi:10.1302/0301-620X.100B2.BJJ-2017-0531.R2.
Tarabichi M, et al. J Bone Joint Surg Am. 2018;doi:10.2106/JBJS.17.00434.
Tirumala V, et al. J Arthroplasty. 2021;doi:10.1016/j.arth.2020.08.065.
Torchia MT, et al. J Arthroplasty. 2019;doi:10.1016/j.arth.2019.03.029.
van Dijk EL, et al. Trends Genet. 2014;doi:10.1016/j.tig.2014.07.001.
Wong L-JC. Next-Generation Sequencing Analyses of the Whole Mitochondrial Genome. In: Wong L-JC, ed. Next Generation Sequencing: Translation to Clinical Diagnostics. New York: Springer; 2013:203-219.