Spaceflight may be linked to somatic mutations, increased risk for cancer, heart disease
Click Here to Manage Email Alerts
Spaceflight may place astronauts at increased risk for developing mutations that can lead to cancer and heart disease, according to a study published in Nature Communications Biology.
“Deep-space missions such as to the moon or Mars will be associated with exposure to a harsher radiation environment, as astronauts will no longer remain within the Earth’s protective geomagnetic field,” lead author Agnieszka Brojakowska, BA, associate researcher in the lab of senior author David Goukassian, MD, PhD, professor of medicine (cardiology) in the Cardiovascular Research Institute at Icahn School of Medicine at Mount Sinai, told Healio. “Radiation-induced carcinogenesis and cardiovascular disease are primary concerns associated with prolonged spaceflight, so NASA aims to optimize current shielding technologies to minimize radiation exposure.”
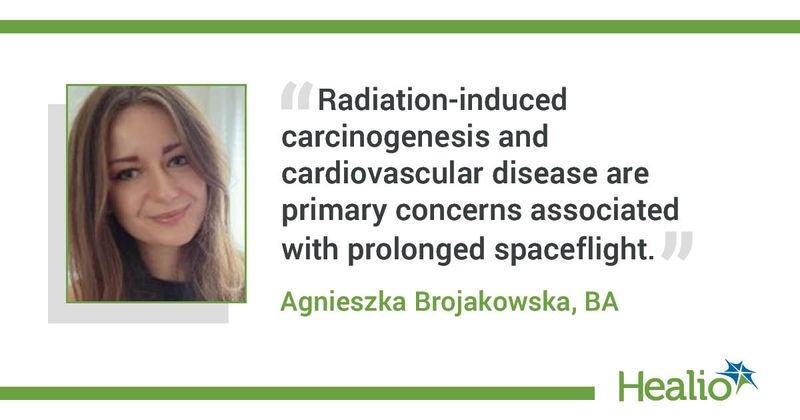
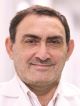
Brojakowska and Goukassian spoke with Healio about how the experience of spaceflight may engender these increased health risks and how they should be prevented going forward.
Healio: How did you identify somatic mutations in the astronauts/participants in your study?
Brojakowska: Peripheral blood mononuclear cells (PBMCs) isolated from 14 astronauts at 3 days after return from their respective shuttle missions (median, 12 days) were sequenced to identify somatic mutations. To assess clonal hematopoiesis in this cohort of astronauts, we performed deep-targeted and error-corrected DNA sequencing using an Archer core myeloid panel (ArcherDx Inc.) to focus on the enrichment of 37 genes involved in clonal hematopoiesis and myelodysplastic syndromes. We analyzed sequencing data using Archer Analysis software. After cleaning and alignment of reads, variants were called by determining allele frequency at a per-variant resolution in reference to a normal data set (sequence data from seven young, healthy individuals) to ensure the detection of true variants. Variants were filtered by allele frequency and Genome Aggregation Database (gnomAD) frequency and annotated using ClinVar and COSMIC databases.
Healio: What is the association between mutations in clonal hematopoiesis of indeterminate potential driver genes and cancer risk?
Brojakowska: Clonal hematopoiesis of indeterminate potential (CHIP) refers to the acquisition of somatic mutations in hematopoietic stem/progenitor cells (HSPCs) without known neoplasia. Most somatic mutations in HSPCs occur in genes involved in epigenetic regulation (DNMT3A, TET2, ASXL1, etc.), DNA damage response (TP53, PPM1D) or regulatory tyrosine kinases (JAK2) and may confer a premalignant condition in these cells. Under selective pressures, subclinical clonal expansion of known leukemogenic driver mutations may drive malignant transformation. Over the last decade, studies have shown CHIP is associated with an increased risk for hematologic and solid malignancies, and overall poorer health outcomes. At a functional level, studies have shown loss-of-function mutations in drivers such as TET2 may promote chronic inflammation; however, further studies are needed to elucidate how CHIP may affect cancer development and therapeutic response.
Healio: How is radiation exposure involved?
Brojakowska: In therapy-related clonal hematopoiesis (t-CH), exposure to genotoxic stress with chemotherapy or radiation therapy is associated with a higher frequency of TP53 and PPM1D mutations. Specifically, studies of clonal hematopoiesis in cohorts of patients with therapy-induced m yelodysplastic syndrome (MDS) compared with primary MDS showed clones harboring such mutations thrive under selective pressure. Similarly, mice transplanted with TP53-mutant bone marrow did not possess clonal advantage following transplant but showed clonal outgrowth following irradiation. These findings suggest that radiation exposure presents a favorable selective pressure for clonal outgrowth compared with cells with normal “healthy” DNA. Additionally, radiation exposure may also be a primary driver of somatic mutation acquisition within HSPCs, which suggests radiation may affect both clonality and temporal dynamics of mutation acquisition.
Healio: The astronauts in your study participated in short space shuttle flights. What might this mean for astronauts who go on longer flights?
Brojakowska: Considering we detected small clones in known clonal hematopoiesis-driver genes in young [median age, 42 years] astronauts following relatively short shuttle flights, we may assume longer missions will be associated with greater exposure to selective pressures (ie, microgravity, increased radiation exposure time and dose, etc.) that will affect clonal stability and may accelerate malignant transformation. The effect an isolated spaceflight stressor such as space radiation has on clonality is unknown, as is how this may be modified with combined stressor exposure (eg, radiation and microgravity); however, in the context of therapy-related CHIP, we know exposure to radiation therapy increases acquisition of mutations in DNA damage response genes such as TP53, which in the context of cardiotoxicity has been shown to promote an inflammatory neutrophil phenotype that perturbs cardiac dysfunction. Further work is required to determine whether quality and radiation exposure rates would accelerate clonal instability and what downstream mechanisms may be targeted for developing biological countermeasures.
Healio: Based on these findings, should NASA consider reducing radiation exposure for astronauts? How might this be achieved?
Brojakowska: Although occupational countermeasures previously limited time spent in space, with deep-space missions we must expand this to include understanding and possibly predicting solar particle events (SPEs) and galactic cosmic ray (GCR) exposure to minimize extravehicular activities and/or deploy additional shielding methods, which may work for SPEs but not for GCR.
Current mitigation techniques are focused on engineering countermeasures (structures or tools to shield from radiation) to minimize exposure, which involves using hydrogen-rich shielding such as polyethylene on the International Space Station. However, these must be optimized to consider the penetrative force of high-energy protons and heavy ions from SPEs and GCR. Although considerations for thicker metal shields or deployment of electrostatic radiation shields may address these concerns, they also pose a limitation due to the sheer weight and occupancy of such systems.
Beyond direct technologies, NASA can also consider using prophylactic medications, for example, enhancing endogenous DNA repair capacity and/or promoting senescence of cells that cannot be repaired efficiently. This will augment physiological mechanisms known to contribute to disease development, such as targeting DNA repair response or, possibly, inflammatory pathways.
Healio: Is there anything else you’d like to add on this subject?
Goukassian: Through these studies, we have demonstrated the potential to assess the health risk of space flight among astronauts. What is important now is to conduct longitudinal retrospective and well-controlled prospective studies involving a large number of astronauts to see how that risk evolves based on continued exposure, and then compare that data against their clinical symptoms, imaging and lab results. These new studies will enable us to make informed predictions of which individuals are more likely to develop disease based on the phenomena we are seeing.
For more information:
Agnieszka Brojakowska, BA, can be reached at Yale School of Medicine, 333 Cedar St., New Haven, CN 06510; email: agnieszka.brojakowska@yale.edu.
David Goukassian, MD, PhD, can be reached at Icahn School of Medicine at Mount Sinai, One Gustave L. Levy Place, Box 1014, New York, NY 10029; email: david.goukassian@mssm.edu.