Detailed history, exam lead to effective neuro-ophthalmic imaging, accurate diagnosis
It is necessary for referring clinicians to provide maximal information regarding the patient’s presentation.
Click Here to Manage Email Alerts
Appropriately diagnosing and managing patients with neuro-ophthalmic disease can be challenging and rewarding to both you and the patient. The nature of the disease course, urgent or emergent, will dictate which neuro-imaging study will deliver the most essential information in a timely manner. Combining knowledge of neuro-ophthalmic anatomy with the proper imaging technique will allow for precise detection of lesions involving the afferent and efferent visual system.
The most common clinical indications for the use of neuro-imaging are: visual loss, unilateral or bilateral visual field defects and scotomas, anisocoria or ptosis, proptosis, diplopia, ophthalmoplegia, oscillopsia, optic nerve anomalies and pupillary defects, according to Johnson and colleagues. In general, magnetic resonance imaging (MRI) is the modality of choice for imaging suspected neuro-ophthalmic disease processes, while computed tomography (CT) is more appropriate for evaluating bone in cases of trauma and osseous lesions of the bony orbit or optic nerve calcifications. As the referring clinician, it is necessary to provide maximal information with regard to the patient’s clinical presentation.
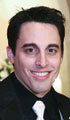
Michael DelGiodice
Prior to modern neuro-imaging techniques, the assessment of ocular structure was limited to direct ophthalmologic visualization. With the advent of early radiography (x-ray tomography) a limited application of indirect visualization was enabled with the use of electromagnetic radiation to view non-uniform material, such as the human body. Hoffer defines tomography as a method of producing a three-dimensional (3D) image of the internal structures of a solid object by observing and recording the differences in the effects of the passage of waves of energy impinging on those structures.
Computed tomography

Attefa Sultani
CT involves the use of electromagnetic radiation and digital geometry processing to generate a 3D image of the inside of an object by a series of plane cross-sectional images along an axis. CT is both cost and time efficient in providing vital information with respect to the brain, orbit and bone. It is the imaging modality of choice for evaluating acute hemorrhage, calcification and bony abnormalities. CT scanning is painless, noninvasive, accurate and less sensitive to patient movement than MRI. Unlike MRI, CT can be performed with implanted medical devices of any kind and provides real-time imaging during invasive procedures such as needle biopsy, according to Hoffer.
Disadvantages of CT include ionizing radiation, beam-hardening artifacts and iodinated contrast-induced allergic responses, and nephrotoxicity. Soft-tissue details in areas such as the brain, internal pelvic organs and joints (such as knees and shoulders) can often be better evaluated with MRI. Furthermore, CT imaging is not indicated in children, patients who are pregnant or those who weigh more than 450 pounds (Hoffer).
CT imaging is usually performed using one of the three axes: transaxial, coronal or sagittal (an axis a line from the bottom of the feet to top of the head):
-
The transaxial plane divides the body into superior and inferior parts. It is perpendicular to the coronal and sagittal planes. Head CTs are usually taken with a transaxial approach, extending from the foramen magnum to vertex.
-
The coronal plane is any vertical plane that divides the body into frontal and dorsal sections. It shows left and right, superior and inferior as seen from the front. It is often useful for CT scans of the sella and pituitary gland and paranasal sinus areas.
-
The sagittal plane is a vertical plane that passes from ventral (front) to dorsal (rear), dividing the body into right and left halves. It shows anterior to posterior, and superior and inferior as seen from the side.
CT imaging can be done with or without contrast. CT contrast agents, also referred to as dyes, are used to highlight specific areas within organs, blood vessels and tissues. According to Hoffer, these pharmaceutical agents may consist of iodine, barium, barium sulfate and gastrografin. They can be administered in four different ways: intravenous injection, oral administration, rectal administration and inhalation.
Intravenous contrast is most often used to highlight blood vessels and enhance tissues within the brain, spine, liver and kidney. Typically, iodine is injected into the bloodstream. As it circulates through the body, the CT’s x-ray beam is generally weakened as it passes through blood vessels and organs that have taken up the contrast and show up as white areas on the CT images. When the test is finished, the kidneys and liver quickly eliminate the contrast from the body.
Magnetic resonance imaging
MRI makes use of nuclear magnetic resonance technology, in which magnetic nuclei absorb and re-emit electromagnetic radiation in a magnetic field. While inside the magnetic field of an MRI, the resonance frequency is used to flip the spin of the protons then realign them to thermodynamic equilibrium in a matter of seconds. Thus, the proton’s spin density, relaxation time and flow, and spectral shifts are employed to construct images, according to Weishaupt and colleagues.
MRI provides good contrast between the different soft tissues of the body, making it the procedure of choice for imaging the brain and orbit. Unlike CT, MRI is able to image in any plane, creating axial images, and uses no ionizing radiation, making it a safe procedure and modality of choice in pregnancy, children and those with cardiac or renal dysfunction. Specifically, MRI is advantageous in diagnosing early-onset cerebrovascular accident (CVA), multiple sclerosis (MS), tumors and infection of the brain and pituitary gland, visualizing torn ligaments and diagnosing tendonitis. The table summarizes the differences between CT and MRI.
According to Weishaupt and colleagues, disadvantages of MRI include patients with a previous history of metallic foreign body, cochlear implants, cardiac pacemakers and people who are morbidly obese. MRI typically lasts from 20 to 90 minutes and can be difficult to sit through given the anatomic location of the image. Lastly, given the use of gadolinium, those who have active or undiagnosed kidney disease can develop an incurable condition called nephrogenic systemic fibrosis, causing hardening of the skin and joints.
MRI can be performed using different signal intensities. The signal intensity is determined by four parameters: proton density, T1 relaxation time, T2 relaxation time and flow. Proton density refers to the concentration of protons in a particular tissue in the form of macromolecules. The T1 and T2 relaxation times refer to the resting state of the protons following excitation via the initial radiofrequency pulse. Lastly, flow represents the effect of lost signal from arterial blood flow (Weishaupt and colleagues).
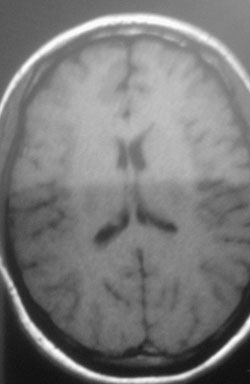
Axial T1-weighted MRI.
Images: DelGiodice M
A T1-weighted scan is the longitudinal relaxation time, representing the time it takes tissue to recover from a radiofrequency pulse. It is the standard scan clinically used to differentiate fat from water and white matter from gray matter in the brain. With T1 weighted images, fluid is very dark, water-based tissue is grey, and fat-based tissues are very bright. Due to the short repetition time, (TR), this scan can be run very fast allowing the collection of high resolution 3D datasets and allow for better resolution of white and grey matter, according to Weishaupt and colleagues.
A T2-weighted scan represents the spin-spin relaxation time, the time the signal lasts after giving a radiofrequency pulse. With T2-weighted images, fluids have the highest density, with water appearing light and fat-based tissue appearing dark.
A T2 star is a weighted scan that uses a gradient echo (GRE) sequence with long TE (echo time) and long TR. GRE sequence is most often used in suspected cases of micro-hemorrhage in the brain. These images provide good contrast between gray and white matter in the brain, iron-laden tissues and venous vessels, according to Weishaupt and colleagues.
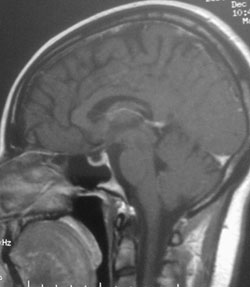
Sagittal T1-weighted MRI revealing an empty sella, with contrast.
Inversion recovery, fluid attenuated inversion recovery (FLAIR) and standard short T1-inversion recovery (STIR) are magnetization preparation techniques employed to suppress certain anatomic tissue. FLAIR sequence produces heavily weighted T2 images with suppression of cerebrospinal fluid, allowing for more sensitive resolution in suspected demyelination or lesions anatomically close to the ventricles. STIR sequence employs spin echo completed by a previous 180-degree inversion pulse. The advantage of STIR is that it can be used with T1- or T2-weighted images with fat signal suppression. Other pulse sequences of MRI include magnetization transfer contrast, diffusion-weighted, echo planar, perfusion and functional (Weishaupt and colleagues).
Neurovascular imaging
The ability to visualize the neurovascular anatomy involves expressing the blood vessels and suppressing the surrounding structures. In catheter angiography (CAG) and computerized tomography angiography (CTA) an intravascular contrast agent is injected to create a detailed analysis of the vascular system, while magnetic resonance angiography (MRA) expresses vessels by dampening and suppressing the surrounding anatomic structures.
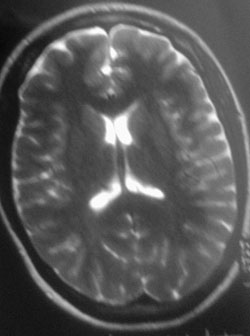
Axial T2-weighted MRI.
Both CTA and MRA are successful as noninvasive procedures for studying the vasculature system. However, for the coronary arteries, CTA is much more effective in visualizing the lumen and detecting secondary structural changes from atherosclerosis, according to Schneider and colleagues. Furthermore, CTA is less expensive, more accessible, less time consuming and applicable for patients with implantable devices (that is, pacemakers and certain aneurysm clips) as well as those with claustrophobia and difficulty breathing.
With MRA, a low dose of gadolinium contrast is injected into a vein, and images are acquired during the first pass of the agent through the arteries. The images obtained, unlike CAG or CTA, do not display the lumen of the vessel, but rather the blood flowing through the vessel, allowing for visualization of intravascular abnormalities. In addition, MRA is the modality of choice for children, pregnant women and those with cardiac or renal dysfunction (Schneider and colleagues).
Catheter angiography is a form of angiography that provides images of blood vessels in the body by inserting a tube into a large artery and threading it through the circulatory system, where a contrast dye is injected. As the contrast spreads throughout the arterial system, a series of radiographs is taken. As the dye exits via the venous system, a second series of radiographs is taken. For some applications, this method may yield greater resolution when compared to CTA and MRA. Furthermore, CAG allows for endovascular treatment to be performed immediately based on the findings. However, major complications in CAG do exist: CVA, allergic reaction to the anesthetic or contrast medium, blockage or damage to one of the access veins in the leg, or thrombosis and embolism formation. Minor complications include bleeding or bruising at the site where the dye is injected, according to Schneider and colleagues.
The most common uses of this procedure are to image atherosclerotic disease in the head and neck, identify intracranial aneurysm, evaluate disease in the kidney and aorta, identify the source of occult bleeding and to help prepare for vascular surgery. Limitations of CAG include imaging in children, pregnancy, those with cardiac and impaired kidney function, history of allergy to the contrast agent and those who have a tendency to bleed.
Case 1
A 69-year-old male presented with acute-onset diplopia and a droopy right eyelid lasting 1 day. He denied associated neurologic symptoms. His past medical history was positive for type 2 diabetes mellitus, hypertension (HTN) and high cholesterol, which are being treated with medications. On examination, his best corrected visual acuity (BCVA) measured 20/30 OU, and gross observation revealed mild ptosis in the right eye. Ocular motility revealed limited adduction and elevation in the right eye and full movement in the left eye. In primary gaze, the right eye was in a down and out position, and the left eye was centered. Forced duction testing was negative, and no evidence of aberrant regeneration existed. In bright illumination, the right pupil measured 6 mm and was minimally reactive; the left eye measured 3 mm in bright illumination and had normal reactivity. Intraocular pressure was 12 mm Hg OU. Anterior segment evaluation and dilated fundus examination was unremarkable. Given the above clinical scenario, the most likely diagnosis is incomplete relative pupil-sparing third-nerve palsy in the right eye.
The critical signs of this case include incomplete ophthalmoplegia with ipsilateral ptosis and relative-pupil involvement in the right eye (pupil is partially dilated and sluggishly reactive to light). Partial and complete oculomotor palsies (OMPs) without pupil involvement are most aptly attributed to ischemic processes secondary to vasculopathic disease such as diabetes and hypertension; the clinical course will be the limiting factor in determining the urgency and extent of neurologic and vascular imaging.
It is prudent to examine these patients a few times during the first 2 weeks and monthly thereafter to determine pupil involvement and resolution, as late-onset pupil-involving OMPs have been recorded up to 14 days after the initial presentation. According to Capo and colleagues, 100% of OMPs caused by ischemic events resolved within 4 months of initial presentation, thus a non-resolving, pupil-absent OMP should be scanned urgently to exclude a compressive lesion within the cavernous sinus or referred for lumbar puncture to exclude lymphoma. On the contrary, pupil-involving partial or complete OMPs are 86% to 100% of the time the result of intracranial aneurysm most commonly involving the posterior communicating artery and less commonly the internal carotid artery within the cavernous sinus and basilar artery, according to Capo and colleagues. This is sufficient evidence to warrant emergent neuroimaging and angiography.
This case most aptly demonstrates a partial pupil-involving OMP that may be an early sign of aneurysmal compression. Other than the pertinent ophthalmologic signs, the patient is neurologically intact, making a potential ruptured aneurysm unlikely. However, given the high risk of both mortality and neurologic morbidity, potential aneurysmal expansion carries the chance of rupture and subarachnoid hemorrhage. Thus, given the fact that the causative aneurysm usually measures 4 mm or greater in diameter, the modality of choice is emergent CT/CTA. In the event that the results of the imaging are negative and the clinical suspicion of aneurysm is high, combined contrast-enhanced MRI/MRA should be ordered, as it is superior in viewing the full course of the third nerve from its exit at the midbrain to its insertion through the superior orbital fissure and it is unique in detecting intraluminal thrombus. Lastly, if the results are still negative, cerebral angiography should be performed.
Case 2
A 50-year-old female presented with complaints of constant double vision and painful red eyes for 1 week. Her medical history was unremarkable. She denied neurologic symptoms. On examination, her BCVA was 20/20 OU. Gross observation revealed mild proptosis in the right eye with resistance to retropulsion. Hertel measurement with Hirschberg assessment revealed inferior and nasal displacement in the right eye and normal alignment in the left eye. Ocular motility testing revealed a mild elevation and abduction deficit in the right eye and normal motility in the left eye. Pupil testing was normal in both eyes. IOP was 15 mm Hg in primary gaze and 21 mm Hg when measured in upgaze in the right eye and 15 mm Hg in primary and upgaze in the left eye. No other eyelid abnormalities were observed. Interpalpebral aperture measurement was 15 mm OD and 12 mm OS. There was no evidence of lid lag in downgaze or nystagmus in either eye. Anterior segment evaluation revealed mild lid retraction and moderate diffuse conjunctival hyperemia in both eyes. Dilated fundus evaluation was unremarkable. Given the above clinical scenario, the most likely diagnosis is thyroid eye disease (TED).
TED, thyroid-related ophthalmopathy and Graves’ orbitopathy are all relative terms used to describe the ophthalmologic findings associated with Graves’ disease, an autoimmune disorder targeting the orbit, periorbit and thyroid gland, according to Douglas and colleagues. Thyroid eye disease has a biphasic course consisting of active inflammation with lymphocytic infiltration and an accumulation of glycosaminoglycans within the orbital tissue, followed by a period of progressive fibrosis. The first phase most often targets soft tissue leading to orbital fat expansion and can last 1 to 5 years. Following the acute phase, a second phase, consisting of progressive fibrosis, results in extraocular motility constriction.
Thyroid eye disease can occur in the presence or absence of systemic thyroid abnormality, with the majority of patients being hyperthyroid and a much smaller percent presenting as hypothyroid or euthyroid, according to Lee and colleagues.
The critical signs of TED include unilateral or bilateral proptosis, restricted ocular motility, resistance to retropulsion of the globe, eyelid retraction and lid lag in downgaze, all of which may play an integral role in the incidence of ocular morbidity and decreased vision, according to Kunimoto and colleagues. There are many potential causes of visual loss in the setting of TED, the two most common being keratopathy and optic nerve compression.
While the clinical presentation is highly indicative of TED, the acute onset of symptoms, lack of lid retraction, along with absent thyroid disease warrants urgent noncontrast CT of the head and orbits along with three blood tests: thyroid-stimulating hormone, total T4 and thyroid-stimulating immunoglobulins. CT will rule out mimickers, as well as demonstrate proptosis, extraocular muscle enlargement that is tendon sparing, increased orbital fat volume and enlargement of the superior ophthalmic vein, all of which are unique to TED (Lee and colleagues).
Case 3
An 82-year-old female presented for her annual eye exam without visual complaints but did report that her daughter had mentioned 1 week ago that one pupil was larger than the other. Her ocular history was positive for blepharoplasty surgery 10 years prior. She admitted to being a social drinker and smoker for the past 50 years. Her medical history was positive for a mild case of chronic obstructive pulmonary disorder and osteoarthritis for approximately 30 years, which are being treated. Her family history was contributory for CVA, HTN and high cholesterol. She denied neurological symptoms. On examination, her BCVA measured 20/50 OU. Ocular motility testing was full and smooth in both eyes. Pupils in dim illumination measured 5 mm OD and 3 mm OS, and in bright illumination pupils measured 3 mm OD and 2 mm OS. Anterior segment evaluation revealed mild eyelid asymmetry and mild cataracts but was otherwise unremarkable. Interpalpebral aperture measurements were 12 mm OD and 10 mm OS. IOP measured 11 mm Hg OU. Given the above clinical findings, a left Horner syndrome (HS) was suspected. Subsequently, one drop of 1% hydroxyamphetamine was instilled in each eye. After 45 minutes, both pupils dilated, confirming a preganglionic lesion. Dilated fundus evaluation was unremarkable.
Horner syndrome is a condition related to sympathetic innervation and is described by Walton and Buono as a triad of signs consisting of pupillary miosis, mild upper lid ptosis, and facial and skin anhydrosis. Diagnosing Horner syndrome is based on clinical signs followed by confirmatory pharmacologic testing. Topical apraclonidine in either 0.5% or 1% concentration has generally replaced topical cocaine in confirming the presence of HS and is followed by 1% hydroxyamphetamine to aid in localizing the pathway of the sympathetic chain (Freedman and Brown).
Except for rare emergencies, as in acute HS suggesting carotid artery dissection in which neuroimaging should be performed immediately, or, in cases of chronic HS with previous incidence of trauma to the neck, upper spine or chest in which no further diagnostic work-up is necessary, localization of the lesion with 1% hydroxyamphetamine should be performed immediately prior to neuroimaging (Trobe). In subtle cases that do not appear emergent, confirmation of the presence of Horner’s syndrome should be immediate and confirmed with either 0.5% or 1% topical apraclonidine; the Horner’s pupil will dilate. The patient should then return the following day for localization of the lesion with 1% hydroxyamphetamine.
Determining which imaging modality should be employed is based on clinical features, symptoms and pharmaceutical localization. Because carotid artery dissection is a life-threatening cause and can occur in the absence of pain, a vascular study such as MRA or CTA should be obtained in all suspect cases of HS along with CT or MR imaging studies of the brain and chest.
This case demonstrates a preganglionic Horner’s syndrome due to left pupillary dilation upon 1% hydroxyamphetamine instillation. This indicates either first- or second-order neuron involvement. First-order lesions typically localize to the hypothalamus, brainstem and spinal cord, whereas second-order lesions involve the cervical spine, brachial plexus, lung region, subclavian artery and thyroid gland (Reed and colleagues). Given the patient’s history, the HS has likely been present for a minimum of 1 week. Within the first 2 weeks of acute HS there is significant risk of hemispheric stroke due to carotid artery dissection with middle cerebral artery involvement, thus, this patient should be managed on an emergency basis (Biousse and colleagues).
The preferred method of imaging includes MRI of the chest, MRI with and without contrast of the head and neck with diffusion weighted sequences, and CTA of the head and neck. MRI of the chest will exclude a tumor located at the lung apex. MRI with and without contrast of the head and neck will help rule out stroke, tumors and demyelination. CTA is advantageous as it is a noninvasive technique to evaluate for the presence of carotid artery dissection, subclavian artery aneurysm and arteriovenous malformation. It is important to remember that specific requisition should be made with regard to the anatomic location of interest either noted during the history or in the physical exam.
Case 4
A 77-year-old male presented with a shadow on the left side of his vision for 1 week and a brief episode of vision loss that lasted 5 minutes. He had a history of HTN and high cholesterol controlled with medication for the past 20 years. He denied symptoms of both neurologic disease and temporal arteritis. BCVA measured 20/25 OD and 20/30 OS. Ocular motility testing was full and smooth OU. Confrontation visual field testing (CFVF) revealed a nasal defect in the right eye and temporal defect in the left eye. Slit lamp biomicroscopy was normal with the exception of 1+ nuclear sclerotic cataract in both eyes. The patient underwent Humphrey 24-2 SITA testing (Carl Zeiss Meditec, Dublin, Calif.), which confirmed a left incomplete congruous homonymous hemianopia (HH). Dilated funduscopy was noncontributory. Given the above clinical findings, cerebrovascular accident (CVA) with associated amaurosis fugax was the most likely etiologic process.
This case represents a medical emergency, as it consists of both acute HH and amaurosis fugax. HH is characterized as a visual field defect that most aptly precludes occipital lobe involvement. Among those with HH, the overwhelming majority of cases are secondary to ischemic CVA, followed by hemorrhagic CVA, head trauma, brain tumor, neurosurgical procedures and multiple sclerosis. According to Zhang and colleagues, the most common cause of homonymous hemianopia in an elderly patient with microvascular disease is occipital stroke.
Amaurosis fugax, literally translated as “fleeting blindness,” is a relative term characterizing transient visual loss. Clinically it can be divided into four categories based on symptoms and associated etiology. A high index of suspicion should be undertaken as the onset of visual loss, length of symptoms, recovery and presence or absence of pain can greatly vary. The best approach to management includes a detailed history, physical exam and vascular imaging of both carotid and cardiac vessels. According to Burde, at 7 years after the onset of symptoms, 14% of patients with isolated amaurosis fugax suffered a stroke.
In this case, given the lack of associated neurologic symptoms, the most likely etiology is ischemic stroke within the occipital lobe along with embolism or atheroma of the carotid and cardiac vasculature. This patient requires immediate referral to the emergency room for noncontrast CT of the head, along with ultrasound and blood flow analysis of the carotid arteries and urgent neurologic evaluation. While the patient denied neurologic symptoms, immediate noncontrast CT scanning can rule out a life-threatening intracranial hemorrhage (such as subarachnoid hemorrhage). If the CT is unremarkable, cranial MRI with and without contrast may reveal the presence of brain infarcts related to the degree of carotid artery stenosis.
The next step would be to order a carotid duplex scan and echocardiogram to evaluate the integrity of both the carotid and cardiac vessels. Provided high-grade stenosis is detected on duplex scanning, carotid endarterectomy could be employed. If the stenosis was mild to moderate and the clinical suspicion of nonstenosis related pathology was high, MRA or CAG of the neck may be required.
Case 5
A 33-year-old female presented with acute headaches, transient visual blur and pulsatile tinnitus. She denied neurologic symptoms, took no medication and had an unremarkable systemic history. Best corrected visual acuity measured 20/20 OU. Externals and anterior segment examination was unremarkable. Color vision testing was normal in both eyes. Fundus examination revealed optic disc edema, absent spontaneous venous pulsation and nerve fiber layer hemorrhage in both eyes. Visual field testing showed an enlarged blind spot in both eyes. Given the above clinical findings, this case most likely reflects presumptive papilledema.
Papilledema is a term used to describe bilateral optic disc edema and intracranial hypertension (ICH). According to Wang and colleagues, the clinical features of ICH include obesity, blurred vision, seizure, pulsatile tinnitus, bilateral optic disc edema and diplopia due to sixth nerve palsy. With many of the features overlapping, a thorough review of systems, neuroimaging and cerebrospinal fluid (CSF) evaluation will help distinguish idiopathic ICH from its secondary forms. Neuroimaging studies are of value in determining the presence of structural abnormalities most often found in secondary ICH, and lumbar puncture allows for measuring both pressure and cellular constituents of the CSF. Neuroimaging findings consistent with ICH may include: empty sella, smooth walled venous stenosis, optic nerve sheath dilation, flattening of the posterior globe and enhancement of the optic disc (Friedman and Jacobson). For a diagnosis of primary ICH, neurodiagnostic studies should be negative with the exception of increased CSF (more than 250 mm of water on opening pressure), according to Marcels and Silberstein.
With regard to ophthalmologic evaluation, the optic nerves may or may not reveal the presence of edema. The most common features of optic nerve involvement may include: blurring of the disc margins, peripapillary halo, venous congestion and tortuosity, retinal nerve fiber layer hemorrhages, cotton-wool spots and intraretinal exudates (Marcels and Silberstein). Cases of primary ICH without disc edema are not well defined but may account for 5% of all cases seen in neuroophthalmology practice, according to Digre and Friedman. These patients tend to be diagnosed later in the disease process and do not respond to treatment as well as those with papilledema. Such cases may be amenable to MR venography (MRV) to rule out venous sinus thrombosis as a secondary cause.
Given the above clinical findings, emergent MRI with and without contrast of the brain and orbits must be ordered first to rule out intracranial pathology (that is, ventricular dilation, hydrocephalus and mass lesion). A normal MRI should be followed by lumbar puncture to evaluate the cerebrospinal fluid (CSF) constituents and record the opening pressure. A “clean” MRI and opening pressure of greater than 250 mm of water would necessitate a treatment course of low-sodium-weight management with weight loss of 5% to 10% along with 250 mg to 500 mg of oral acetazolamide dosed two to four times daily depending on the amount of baseline vision loss and the degree of papilledema, according to Lee and colleagues.
Case 6
A 70-year-old male presented with acute-onset painful proptosis, chemosis, redness, diplopia and associated right facial pain following a motor vehicle accident 2 days prior. BCVA measured 20/200 OD and 20/20 OS. Gross observation revealed complete ptosis and marked proptosis in the right eye. Hertel exophthalmometry confirmed a 5-mm difference between the right and left eye. Slit lamp biomicroscopy revealed moderate conjunctival chemosis and injection, iris neovascularization and dilated episcleral vessels in the right eye and normal anterior segment in the left eye. CFVF was full in both eyes. IOP was 32 mm Hg OD and 18 mmHg OS. Pupil evaluation revealed a dilated, sluggish pupil in the right eye and normal response in the left eye. Extraocular motility testing revealed complete ophthalmoplegia in the right eye with full and smooth movements in the left eye. Forced duction testing was negative. Dilated fundus evaluation revealed a central retinal vein occlusion in the right eye and normal retina constituents in the left eye. Based on the presentation of acute proptosis, orbital congestion, facial pain and closed-head injury following a motor vehicle accident, a tentative diagnosis of high-flow carotid-cavernous fistula (CCF) was likely.
CCFs represent acquired aberrant connections between the internal or external carotid arteries and the cavernous sinus. They can be categorized as either high-flow, direct CCF or low-flow, indirect CCF. The most common cause of high-flow CCF is head trauma with secondary skull fracture causing rupture of the internal carotid artery within the cavernous sinus, according to Glaser. The symptoms of high-flow CCF are typically acute in nature, occurring within days to weeks, and include: ocular bruit, proptosis, redness and chemosis of the conjunctiva, diplopia, blurred vision and orbital pain (Sanders and Hoyt). In addition to the external examination, IOP elevation may occur as a result of raised episcleral venous pressure or retinal ischemia-induced neovascular glaucoma. Furthermore, dilated fundus examination may yield intraretinal hemorrhages, venous stasis, optic disc edema, vitreous hemorrhage, central retinal vein occlusion and proliferative retinopathy, according to Lee and colleagues.
While CT, MRI, CTA, MRA and CAG are all capable of diagnosing the condition, patients who are highly suspicious of high-flow CCF must receive immediate referral for screening with contrast-enhanced CT of the head and orbits to rule out the rare but fatal incidence of intracranial hemorrhage and epistaxis. CT imaging is also both cost effective and efficient in visualizing the most common radiographic findings of CCF, which include: enlargement of the cavernous sinus, extraocular muscle thickening, protrusion of the globe and superior ophthalmic vein dilatation (Weisberg). In absence of intracranial hemorrhage, the patient should be scheduled for immediate CTA or CAG with a neuroradiologist to determine the exact location and size of the fistula. According to Chen and colleagues and Langer and colleagues, CTA outperformed MRA in its diagnostic ability and has proven itself as useful as CAG for detecting carotid cavernous fistulae. However, patients who are highly suspicious for CCF and have negative CTA must undergo CAG.
Case 7
A 41-year-old female presented with decreased vision in the left eye and painful diplopia over the past week. She denied associated neurological symptoms and past medical and family history. On examination, her BCVA measured 20/20 OD and 20/50 OS. Extraocular motility testing revealed a left adduction deficit with contralateral horizontal gaze nystagmus in the abducting eye. On right gaze, forced duction testing was negative. Pupil evaluation was normal in the right eye and positive for a mild afferent defect in the left eye. CFVF was full in both eyes. IOP was 17 mm Hg OD and 15 mm Hg OS. Anterior segment evaluation was unremarkable in both eyes. Ishihara color plates were 14/14 OD and 4/14 OS. Red desaturation testing revealed 100% OD and 50% OS. Humphrey visual field 24-2 SITA testing was full in the right eye and positive for a central scotoma in the left eye. Dilated fundus evaluation was unremarkable. Given the above clinical findings including the patient’s age, progressive vision loss, diplopia, pain on eye movements and signs of optic neuropathy and internuclear ophthalmoplegia (INO), a tentative diagnosis of demyelinating INO and retrobulbar optic neuritis was made.
The diagnosis of optic neuritis is typically made clinically, with MRI being reserved for atypical cases of optic neuropathy associated with prolonged or severe pain, lack of visual recovery, atypical visual field loss and evidence of orbital inflammation. However, as per the Optic Neuritis Treatment Trial, baseline MRI is recommended due the association of optic neuritis with MS (Beck and Trobe). In this setting, CT scanning has a limited role due to poor prognostic and treatment-altering yield. Thus, the imaging modality of choice is MRI of the brain and orbits with and without contrast to rule out demyelinating lesions and structural mimics. According to Lee and colleagues, MRI requisition should indicate the clinical question being addressed, relevant clinical history, physical findings, date and place of previous MRI, and standardized brain protocol. Specifically, thin (1 mm to 3 mm), fat-suppressed, T-2 weighted images through the brain and optic nerves should be ordered to allow for visualization of high-signal intensity white matter changes, along with T-2 weighted, FLAIR images. The T2-weighted images with FLAIR technology allows for CSF suppression and is superior at identifying hyper-intense lesions that indicate active disease.
In conclusion, each case will call for specific neuroimaging techniques. Careful patient history and examination will allow for efficient and effective testing and proper diagnosis.
References:
- Ahsan H, Rafique MZ, Ajmal Fozia, et .l: Magnetic resonance imaging findings in white matter disease of brain, demyelinating and demyelinating white matter. J Pak Med Assoc. 2008;58(2):86-88.
- Beck RW, Trobe JD, Optic Neuritis Study Group. The optic neuritis treatment trial. Putting the results in perspective. J Neuroophthalmol. 1995;15(3):131-135.
- Biousse V, D’Anglejan-Chatillon J, Touboul PJ, et al. Time course of symptoms in extracranial carotid artery dissection. A series of 80 patients. Stroke. 1995;26:235-239.
- Burde RM. Amaurosis fugax. An overview. J Clin Neuroophthalmol. 1989;9(3):185-189.
- Caplan LR, Hertzer NR. The management of transient monocular visual loss. J Neuroophthalmol. 2005;25(4):304-312.
- Capo H, Warren F, Kupersmith MJ. Evolution of oculomotor nerve palsies. J Clin Neuroophthalmol. 1992;12(1):21-25.
- Chaudhary N, Davagnanam I, Ansari S, et al. Imaging of intracranial aneurysms causing isolated third cranial nerve palsy. J Neuroophthalmol. 2009;29:238-244.
- Chen CC-C, Chang PC-T, Shy C-G, Chen W-S, Hung H-C. CT angiography and MR angiography in the evaluation of carotid cavernous sinus fistula prior to embolization: a comparison of techniques. Am J Neuroradiol. 2005;26:2349-2356.
- Cremer SA, Thompson HS, Digre KB, Kardon RH. Hydroxyamphetamine mydriasis in Horner’s syndrome. Am J Ophthalmol. 1990; 110:71-76.
- Digre KB, Friedman DI. An overweight young woman with new headache and normal-appearing optic discs. J Neuroophthalmol. 2010;30:85-89.
- Douglas RS et al. Development of criteria for evaluating clinical response in thyroid eye disease using a modified Delphi technique. Arch Ophthalmol. 2009;127(9):1155-1160.
- Freedman KA, Brown SM. Topical apraclonidine in the diagnosis of suspected Horner syndrome. J Neuroophthalmol. 2005;25:83-85.
- Friedman DI, Jacobson DM. Diagnostic criteria for idiopathic intracranial hypertension. Neurology. 2002;59:1492-1495.
- Glaser JS. Neuro-ophthalmology. Hagerstown, MD: Harper & Row; 1978:336-338.
- Hoffer M. CT teaching manual: a systematic approach to CT reading. New York, NY: Thieme Medical Publishers Inc; 2007.
- Johnson MC, Policeni B, Lee AG, Smoker WR.K. Neuroimaging in ophthalmology. New York, NY: Oxford University Press Inc; 2011.
- Kunimoto, DY, Kanitkar KD, Makar M, et al. The Wills Eye Manual. Office and emergency room diagnosis and treatment of eye disease. Philadelphia, PA: Wolters Kluwer LWW; 2004.
- Langer PD, Reed MD, Turbin RE, Farkas JA. Diagnosis and evaluation of carotid cavernous fistulas and arterio-venous malformations of the cavernous sinus using CT angiography. Invest Ophthalmol Vis Sci. 2004;45:e-abstract 257.
- Lee AG, Brazis PW, Kline LB, Chang DF. Curbside consultation in neuro-ophthalmology: 49 clinical questions. Thorofare, NJ: SLACK Inc; 2009.
- Lee AG, Brazis PW, Kline LB, Chang DF. Curbside consultation in neuro-ophthalmology: 49 clinical questions. Thorofare, NJ: SLACK Inc; 2009.
- Marcels J, Silberstein SD. Idiopathic intracranial hypertension without papilledema. Arch Neurol. 1991;48:392-399.
- Reede DL, Garcon E, Smoker WR, Kardon R. Horner’s syndrome: clinical and radiographic evaluation. Neuroimaging Clin N Am. 2008;18:369-385.
- Sanders MD, Hoyt WF. Clinical Neuro-ophthalmology, Vol. 2, 3rd ed. Baltimore: Williams and Wilkins, 1969:1714-1734.
- Schneider G, Prince MR, Meaney JFM, Ho VB. Magnetic resonance angiography. Techniques, indications and practical applications. Italy: Springer-Verlag Italia; 2005.
- Tabassi A-R, Dehghani A-R, Mosayebi H. Etiology of oculomotor nerve paralysis. J Ophthalmic Vis Res. 2008;1:1.
- Trobe JD. The evaluation of Horner syndrome. J Neuroophthalmol. 2010;30(1):1-2.
- Trobe JD. Searching for brain aneurysm in third nerve cranial palsy. J Neuroophthalmol. 2009;29:171-173.
- Walton KA, Buono LM. Horner syndrome. Curr Opin Ophthalmol. 2003;14: 357-363.
- Wang SJ, Silberstein SD, Patterson S, Young WB. Idiopathic intracranial hypertension without papilledema: a case-control study in a headache center. Neurology. 1998;51:245-249.
- Weisberg LA. Computed tomographic findings in carotid-cavernous fistula. Computed Tomography. 1981;5(1):31-36.
- Weishaupt D, Kochli DV, Marincek B. How does MRI work? An introduction to the physics and function of magnetic resonance imaging. Berlin, Germany: Springer-Verlag Berlin and Heidelberg GmbH & Co. K; 2008.
- Zhang X, Kedar S, Lynn MJ, Newman N, Biousse V. Homonymous hemianopia in stroke. J Neuroophthalmol. 2006;26:180-183.
For more information:
- Michael DelGiodice, OD, FAAO, is a partner at Family Eye Health and Vision Center in Garfield, N.J., and also practices at LCA Vision in Paramus, N.J., and Riverdale Vision Care, Riverdale, N.J. He served a residency in primary care and ocular disease at East Orange/Lyons VA Hospital New Jersey Healthcare System. Dr. DelGiodice can be reached at mdelgiodice@yahoo.com.
- Attefa Sultani, OD, FAAO, is an associate optometrist with Associated Eye Physicians in Clifton and Pompton Lakes, N.J., and also practices at Family Eye Health and Vision Center, Garfield, N.J. She served a residency in ocular disease and special testing at the State University of New York State College of Optometry and Woodhull Medical and Mental Health Center. Dr. Sultani can be reached at attefas@gmail.com.
- Disclosures: The authors have no relevant financial disclosures.