The ABCs of antimicrobial drug resistance in gram-negative bacilli
Some gram-negative bacilli, or GNB, are inherently resistant to certain antibiotics. Others acquire resistance spontaneously due to random mutations, as well as by transference of resistance genes from other bacteria. Resistance genes located on mobile genetic elements can readily move to other bacteria of the same species or even to genetically unrelated bacteria by conjugation (via direct cell-to-cell contact or by a bridge-like connection between two bacterial cells), transduction (via a bacterial virus such as a bacteriophage), or transformation (uptake of naked DNA from the environment). Humans and other animals are also mobile vectors of drug resistance, capable of disseminating resistance genes as they travel worldwide.

Donald Kaye

Mathew E. Levison
If a bacterium carries several genes that encode resistance to different classes of antibiotics, it is called multidrug-resistant (MDR), which is defined as resistance to three or more antibiotic agents from three different antibiotic classes. Since a gene that encodes resistance to one drug class is frequently linked to genes that encode resistance to other classes in these MDR pathogens, use of one type of antibiotic may select for resistance to several other antibiotics, even though the organism was not exposed to these other drugs. The presence of efflux pumps that extrude antibiotics of different classes also contribute to MDR. There are a variety of resistance mechanisms encoded by resistance genes, and there are four main types in GNB (see Table 1).
Resistance to fluoroquinolones
Fluoroquinolone (FQ) resistance has steadily increased in gram-negative bacilli since the 1990s. This has occurred both in community-acquired and health care-associated pathogens worldwide, exceeding 50% in some parts of the world, particularly in Asia.
Resistance of GNB to FQ has usually been attributed to the development of chromosomal mutations that alter FQ-binding sites of two target enzymes — DNA gyrase and topoisomerase IV. The alterations diminish FQ binding ability and thereby the bactericidal action of FQ. Other chromosomal mutations can either decrease the bacterial cell’s outer membrane permeability or increase the activity of the drug efflux pumps.
Plasmid-encoded FQ-resistance genes, which were first reported in 1998, cause low-level FQ resistance by encoding polypeptides, which protect targets from the lethal effects of FQ. A plasmid-mediated variant aminoglycoside acetyltransferase also inactivates ciprofloxacin by acetylating its piperazinyl substituent. Other plasmid-mediated genes encode efflux pumps that extrude FQ. These plasmid-mediated genes confer low-level FQ resistance; but the organisms that carry these plasmids are 100 times more likely to give rise to spontaneous FQ-resistant chromosomal mutants than plasmid-free strains. Low-level resistance, due either to chromosomal mutations or plasmid-encoded genes, accumulates and leads to step-wise higher-FQ resistance when the organism is faced with persistent selection pressure.
FQ resistance has occurred especially in Enterobacteriaceae that also produce a CTX-M–type of extended-spectrum beta-lactamase (ESBL) due in large part to the worldwide emergence of a single clonal group of Escherichia coli, identified by multilocus sequence typing as sequence type 131 (ST131). This organism, which causes predominantly community-onset infections, harbors a broad range of virulence and resistance genes that encode resistance to FQ, sulfonamides and broad-spectrum beta-lactams.
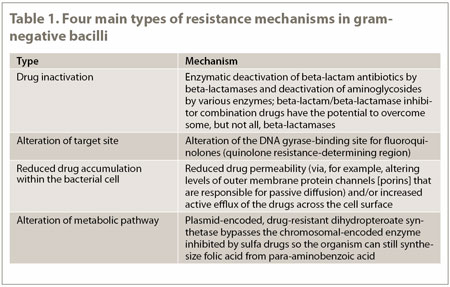
Click the image to enlarge.
The frequency of FQ resistance has varied geographically, not being a problem in some communities, which emphasizes the importance of conducting local surveillance and being aware of the current local antibiotic resistance patterns.
Resistance to beta-lactams
The beta-lactam antibiotics include penicillins, cephalosporins, cephamycins, monobactams and carbapenems. Beta-lactamases (eg, AmpC, ESBLs, carbapenemases) are the primary cause of resistance to beta-lactam antibiotics in GNB.
AmpC
AmpC are clinically important beta-lactamases usually encoded on chromosomes of many Enterobacteriaceae and a few other GNB (Pseudomonas aeruginosa and Acinetobacter spp.), where they mediate resistance to penicillins, cephalosporins, cephamycins and aztreonam. AmpC beta-lactamases are not inhibited by beta-lactamase inhibitors such as clavulanic acid and sulbactam, and are consequently resistant to beta-lactams combined with these beta-lactamase inhibitors. Tazobactam is a moderate inhibitor of AmpC enzymes, although its inhibitory activity is reduced against strains with totally derepressed AmpC beta-lactamases. Ceftolozane, a new cephalosporin that is marketed with tazobactam (Zerbaxa, Cubist Pharmaceuticals), is relatively resistant to hydrolysis by the AmpC beta-lactamase of P. aeruginosa.
Avibactam, a new beta-lactamase inhibitor, blocks the activity of AmpC beta-lactamases. Avibactam also blocks the activity of ESBLs and the serine carbapenemases, including Klebsiella pneumoniae carbapenemases (KPCs) and some OXA carbapenemases, but is not active against the metallo-beta-lactamases: New Delhi metallo-beta-lactamase (NDM), Verona integron-encoded metallo-beta-lactamase (VIM) and imipenemase (IMP) carbapenemases. Avibactam is marketed in combination with ceftazidime (Avycaz, Allergan) and greatly improves the activity of ceftazidime against most species of Enterobacteriaceae that produce AmpC beta-lactamases, ESBLs and some carbapenemases.
AmpC enzymes are inducible and may become constitutively expressed at very high levels (ie, derepressed) due to mutational loss of a repressor control gene. Emergence of derepressed mutants is a problem especially in infections due to Enterobacter aerogenes and E. cloacae, where an isolate initially susceptible to an oxyimino-cephalosporin may become resistant during therapy with these antibiotics.
AmpC beta-lactamases, although typically encoded on the chromosome of many GNB, including Providencia spp., Citrobacter spp., Serratia spp., A. baumannii and Enterobacter spp., may also be carried on plasmids. AmpC encoded on transmissible plasmids now have appeared in bacteria lacking or poorly expressing a chromosomal AmpC gene, such as E. coli, K. pneumoniae and Proteus mirabilis. However, resistance due to plasmid-mediated AmpC enzymes is less common than ESBL production in these organisms in most parts of the world.
Since strains with AmpC genes are often MDR, selection of an effective antibiotic is often difficult. Ceftolozane/tazobactam and ceftazidime/avibactam are therapeutic options, if susceptible. AmpC-producing strains are usually susceptible to carbapenems, and carbapenem therapy has been successful in treating infections due to carbapenem-susceptible strains. However, diminished expression of porin channels through which beta-lactams penetrate the bacterial cell membrane or enhanced efflux in some AmpC-producing strains, as well as the concurrent presence of a carbapenemase, can make such strains carbapenem-resistant as well.
If the isolate is susceptible, FQ or tigecycline are other treatment options. Nitrofurantoin and fosfomycin are oral options for lower urinary tract infections due to susceptible organisms.
ESBLs
GNB resistant to extended-spectrum cephalosporins first emerged in health care settings but now are also spreading in the community. ESBLs have evolved by mutations in genes encoding ampicillin-hydrolyzing beta-lactamases TEM-1 or SHV-1 found in GNB. Multiple mutations that alter the amino acid configuration around the active site in these beta-lactamases have extended the spectrum of beta-lactam antibiotics susceptible to hydrolysis by these enzymes to include oxyimino cephalosporins, as well as aztreonam, but not 7 alpha-methoxy cephalosporins (the cephamycins) or carbapenems. Their hydrolyzing activity, unlike that of AmpC beta-lactamases, is blocked by inhibitors such as clavulanate, sulbactam or tazobactam. However, some TEM-derived ESBLs have acquired resistance to the inhibition of clavulanic acid and sulbactam, but not tazobactam, thereby showing clinical resistance to the beta-lactam/beta-lactamase inhibitor combinations of amoxicillin/clavulanate, ticarcillin/clavulanate and ampicillin/sulbactam. However, they normally remain susceptible to piperacillin/tazobactam.
Another family of ESBLs, CTX-M beta-lactamases, were named for their greater activity against cefotaxime than other oxyimino-beta-lactam substrates. Rather than arising by mutation, they represent examples of plasmid acquisition of beta-lactamase genes.
ESBLs are now a problem in hospitalized patients worldwide. The prevalence of ESBLs among clinical nosocomial isolates varies from country to country and from institution to institution. In the United States, occurrence of ESBL production in Enterobacteriaceae ranges from 0% to 25%, depending on the institution, with the national average being around 3%. Since 2000, CTX-M–producing E. coli O25:H4 sequence type ST131 has emerged worldwide as a significant cause of community-onset urinary tract infections.
In recent years, ESBL-producing MDR A. baumannii (MDRAb) has increased in prominence as a health care-associated pathogen. Primarily affecting hospital ICUs, MDRAb is associated with longer hospitalizations, greater economic cost and increased morbidity, and has been reported in military service members injured in the Middle East. Infection due to MDRAb can occur sporadically, but is more commonly associated with outbreaks. MDRAb infections typically manifest as respiratory (ventilator-associated pneumonia), urinary tract and wound infections (including burn wounds).
ESBLs are often encoded on plasmids. Plasmids responsible for ESBL production frequently carry genes encoding resistance to other drug classes (eg, trimethoprim/sulfamethoxazole, aminoglycosides and FQ). Therefore, antibiotic options in the treatment of ESBL-producing organisms are often extremely limited. Carbapenems are the treatment of choice for serious infections due to ESBL-producing organisms, yet carbapenem-resistant isolates have been reported. ESBL-producing organisms may appear susceptible to some extended-spectrum cephalosporins. However, treatment with such antibiotics has been associated with high failure rates. For organisms producing ESBLs, apparent in vitro sensitivity to cefepime and to piperacillin/tazobactam is common, but both drugs show an inoculum effect, with diminished susceptibility as the size of the inoculum is increased. Strains producing only ESBLs are susceptible to cephamycins and carbapenems in vitro and show little if any inoculum effect with these agents. Treatment options with oral agents may include nitrofurantoin and fosfomycin.
Carbapenemases
Carbapenemases are a diverse group of mostly plasmid-encoded beta-lactamases that degrade carbapenems. They either have serine or zinc at their active sites. They also degrade the oxyimino-cephalosporins and cephamycins. For the most part, beta-lactam inhibitors, such as clavulanic acid, tazobactam and sulbactam, are inactive against carbapenemases. Aztreonam is stable to the metallo-beta-lactamases, but many carbapenemase-producers are resistant to aztreonam owing to other mechanisms. The genes encoding the carbapenemases are frequently associated with other resistant genes (eg, aminoglycosides and fluoroquinolones). Colistin and tigecycline may retain activity against carbapenemase-producing bacteria, but these antibiotics either have significant side effects, are potentially inferior to more conventional therapies, or can be costly.
Ceftolozane/tazobactam, a new beta-lactam/beta-lactamase combination, has potent activity against P. aeruginosa, including various strains resistant to carbapenems, piperacillin/tazobactam and ceftazidime. Its antipseudomonal activity is attributed to ceftolozane’s ability to evade resistance mechanisms, including efflux pumps, reduced uptake through porins and modification of penicillin-binding proteins.
Resistance to polymyxins — colistin and polymyxin B
Polymyxins are often used as drugs of “last resort” for treatment of MDR GNB infections. However, resistance is now emerging.
Resistance to polymyxins, which historically has been due to chromosomal mutations, frequently involves modification of lipopolysaccharide (LPS) in the bacterial cell wall, which reduces the affinity of the drug for its site of action. However, in 2015, the plasmid-encoded resistance gene mcr-1 was described in pigs, raw pork, and a few hospitalized patients in China. The mcr-1 gene encodes a phosphoethanolamine transferase that catalyzes the addition of phosphoethanolamine to lipid A of LPS. Subsequently, a plasmid-encoded mcr-2 gene was described that has 76.7% nucleotide identity to mcr-1 and encodes a similar gene product.
Since its first report, mcr-1 has been detected in bacteria from animals, humans, food and the environment worldwide. mcr-1 is likely to have been present for a while, being detected in bacteria stored for several years. Of greatest concern is the appearance of the mcr-1 gene in MDR bacteria. Resistance to beta-lactams in many mcr-1–positive isolates has been found to be due to ESBLs. ESBL producers usually retain susceptibility to carbapenems. The transfer of the mcr-1 gene to GNB already resistant to all beta-lactams, including the carbapenems, would make infections due to this organism very difficult to treat medically.
An increasing number of such MDR organisms have been described: mcr-1–positive E. coli isolated from a human wound infection in Germany that was a KPC-2 carbapenemase-producer; mcr-1–positive E. coli isolated from a patient’s urine that also carried the blaVIM-1 carbapenemase gene; and mcr-1–positive Klebsiella pneumoniae that produced an OXA-181 carbapenemase.
A French national survey on carbapenemase-producing isolates recovered in 2014 demonstrated a high rate of colistin resistance in K. pneumoniae (6.2%) and E. cloacae (7.7%). However, these resistance rates remain much lower than those observed in Spain (24.7%) and Italy (43%). Several outbreaks of colistin-resistant KPC-producing K. pneumoniae (mainly attributed to the international epidemic clone type ST258) have been reported across Europe (Greece, Hungary, Italy and the Netherlands). A single outbreak of colistin-resistant VIM-1–producing K. pneumoniae has been described in Spain.
No plasmid-encoded mcr-1 gene was identified among these carbapenemase-producing isolates; rather, colistin resistance was found to be due to chromosomal encoded mechanisms.
The few antibiotics available to treat some MDR infections include aztreonam, ceftazidime/avibactam, ceftolozane/tazobactam, polymyxins, tigecycline, nitrofurantoin and fosfomycin, provided the organisms are still susceptible to these antibiotics. However, nitrofurantoin and fosfomycin are only used to treat lower urinary tract infection in the U.S., where IV fosfomycin is unavailable.
Tigecycline, a glycycline, is often considered for treatment of MDR GNB infections. However, even in the absence of resistance, the effectiveness of this agent for treatment of the most serious infections is a concern. Concentrations of tigecycline in the blood are borderline against susceptible GNB, and there is concern that urine concentrations are inadequate. In addition, the FDA issued a safety communication suggesting an increased risk for death with tigecycline relative to other antibiotics used to treat similar infections.
The main driving force behind antimicrobial resistance is the enormous amount of antibiotic in the environment as a consequence of excessive use. Between 2000 and 2010, total global antibiotic consumption is reported to have grown by more than 30%. The increasing use of antibiotics in both human and veterinary practice and their careless discharge, often accompanied by antibiotic resistance genes, into the environment in pharmaceutical-industrial, farm and human waste have contributed to the increasing presence of antibiotics in the environment, which can exert selective pressure on bacterial populations allowing bacteria expressing resistance genes to survive and susceptible bacteria to die off.
Although per capita consumption is generally higher in high-income countries, the greatest increase in antibiotic use has been in low- and middle-income countries, where there has been increasing prosperity leading to an increased demand for antibiotics. Eighty percent of human use is occurring in the community, either prescribed by health care providers or purchased directly without prescriptions. The CDC estimates that 30% of antibiotic prescriptions in the U.S. are unnecessary.
Similarly, increasing prosperity has led to increasing demand for animal protein, which has intensified food animal production and, consequently, greater use of antibiotics for the prevention of infection and growth promotion of these food animals. More than 70% of the antibiotics used in the U.S. are for animal husbandry. Control of antibiotic use will require a concerted effort from physicians, patients, farmers, the pharmaceutical industry and governments.
- References:
- Bradford PA. Clin Microbiol Rev. 2001;doi:10.1128/CMR.14.4.933-951.2001.
- The Center for Disease Dynamics, Economics & Policy. The State of the World’s Antibiotics, 2015. https://cddep.org/sites/default/files/swa_2015_final.pdf. Accessed October 13, 2016.
- Dalhoff A. Interdiscip Perspect Infect Dis. 2012;doi:10.1155/2012/976273.
- Falagas ME, et al. Lancet Infect Dis. 2010;doi:10.1016/S1473-3099(09)70325-1.
- Falgenhauer L, et al. Lancet. 2016;doi:10.1016/S1473-3099(16)00012-8.
- FDA. FDA Drug Safety Communication: FDA warns of increased risk of death with IV antibacterial Tygacil (tigecycline) and approves new Boxed Warning. http://www.fda.gov/Drugs/DrugSafety/ucm369580.htm. Accessed October 13, 2016.
- Fleming-Dutra KE, et al. JAMA. 2016;doi:10.1001/jama.2016.4151.
- Hooper DC. Emerg Infect Dis. 2001;7:337-341.
- Hope R, et al. J Antimicrob Chemother. 2006;doi:10.1093/jac/dkl414.
- Jayol A, et al. Euro Surveill. 2016;doi:10.2807/1560-7917.ES.2016.21.37.30339.
- Johnson JR, et al. Clin Infect Dis. 2010;doi:10.1086/653932.
- Kümmerer K. J Antimicrob Chemother. 2003;doi:10.1093/jac/dkg293.
- Larsson JDG. Ups J Med Sci. 2014;doi:10.3109/03009734.2014.896438.
- Martinez JL. Environ Pollut. 2009;doi:10.1016/j.envpol.2009.05.051.
- Monaco M, et al. Euro Surveill. 2014;doi:10.2807/1560-7917.ES2014.19.42.20939.
- Nalule Y. The Center for Disease Dynamics, Economics & Policy. Exploring Resistance Map: The Rise of Fluoroquinolone Resistance (Part 1). http://www.cddep.org/blog/posts/exploring_resistancemap_rise_fluoroquinolone_resistance_part_1. Accessed October 12, 2016.
- Peirano G, et al. Int J Antimicrob Agents. 2010;doi:10.1016/j.ijantimicag.2009.11.003.
- Pena I, et al. Int J Antimicrob Agents. 2014;doi:10.1016/j.ijantimicag.2014.01.021.
- Poirel L, et al. Lancet. 2016;doi:10.1016/S1473-3099(16)00006-2.
- ProMED-mail.
- Queenan AM, et al. Clin Microbiol Rev. 2007;doi:10.1128/CMR.00001-07.
- Strahilevitz J, et al. Clin Microbiol Rev. 2009;10.1128/CMR.00016-09.
- Wang R, et al. Open Forum Infect Dis. 2016;doi:10.1093/ofid/ofw132.
- Xavier BB, et al. Euro Surveill. 2016;doi:10.2807/1560-7917.ES.2016.21.27.3028.
- Zowawi HM, et al. Sci Rep. 2015;doi:10.1038/srep15082.
- For more information:
- Donald Kaye, MD, MACP, is a professor of medicine at Drexel University College of Medicine, associate editor of ProMED-mail, section editor of news for Clinical Infectious Diseases and an Infectious Disease News Editorial Board member.
- Matthew E. Levison, MD, is a ProMED-mail associate editor and bacterial disease moderator, professor of public health, Drexel University School of Public Health, and adjunct professor of medicine and former chief of the division of infectious diseases, Drexel University College of Medicine.
Disclosures: Kaye and Levison report no relevant financial disclosures.